3.9: Genes and Behavior
- Page ID
- 119641
This page is a draft and under active development. Please forward any questions, comments, and/or feedback to the ASCCC OERI (oeri@asccc.org).
- Describe the field of behavioral genetics.
- Define epigenetics.
- Describe the major ideas that comprise an evolutionary and computational view of the mind.
- Explain the steps involved in how genes make proteins including how gene expression is regulated.
- Describe the types of variation in the genetic code.
- Discuss the ways in which behavior can influence genes.
- Describe gene-environment interactions.
Overview
Genes, in interaction with the environment, have an enormous influence on the organization of minds and behavior. Each species, with its own unique genetic makeup, has an evolved brain structure and functional organization that determines its innate psychological nature. Human nature is one example, but other species possess their own psychological natures as well, determined by their own brain evolution. Wolves have an innate wolf nature, lions have an innate lion nature, hawks have an innate hawk nature, and so on (Koenigshofer, 2010, 2016). The innate psychological nature of each species is the result of the genes affecting brain organization and function that each species carries in its cells, and the complement of genes that each species possesses is a consequence of the evolutionary history of that species. Behavior genetics studies the effects of genes on behavior and on the information processing operations of the brain, as expressed in behavior. It also investigates the influence of environment and behavior on the expression of genes in the organism--a field called epigenetics (see Module 3.14).
The Influence of Genes on Mind and Behavior
Genetic makeup has a large role in determining human behavior. The influence of genes on behavior has been well established in the scientific community. To a large extent, who we are and how we behave is a result of our genetic makeup. While genes do not strictly determine behavior or our mental processes, they play a huge role in what we do and why we do it.
An Evolutionary and Computational View of the Mind
Since we will be talking not only about behavior, but also about the mind, it is best to talk a little bit about what the mind is. Everyone knows something about what the mind is because we each have a mind and we experience our own mental activities such as conscious awareness of our surroundings, feelings, thoughts, and memories each and every moment of our waking lives. You are using some parts of your mind right now as you read this. So, we know the mind as the collection of our own mental processes and experiences.
But there is another way to think about the mind--as a form of biological adaptation, as a collection of information processing solutions to problems of survival and reproduction that have persisted over much of our evolutionary history as a species. On this evolutionary view, the mind is a collection or bundle of information processing "organs" or "modules" each of which has evolved, over our evolutionary history as a species, to process particular kinds of information from the environment in quite specific ways to help us to survive and reproduce our genes. This is a Darwinian or evolutionary model of what the mind is.
This evolutionary model of the mind makes several assumptions (Cosmides & Tooby, 1997): 1) the mind is brain activity (i.e. the mind is what the brain does), 2) the mind/brain of each species, including the human species, has been constructed to take its present day form as a result of evolution by natural selection in that species (therefore, minds just like bodies differ in some ways from species to species, but also like bodies are also similar across species in some ways), 3) this means that the human mind is "hard wired" in many ways by genetic evolution and thus each individual brain comes "hard wired" by genetic information (genes, DNA) which direct brain development from conception onward, 4) this means that each species has its own genetically evolved psychological nature--applied to humans this means that humans are born with an innate human nature, 5) the mind has many different parts that do many different information processing tasks important to survival and reproduction, especially in the evolutionary past of each species, 6) different parts of our minds are localized in different parts of our brains or in different circuits in our brains (localization of function), 7) the human brain is not a general purpose learning machine but a collection of specialized information processing organs or modules that collectively create in our heads a workable model of reality that guides our behavior successfully toward effective adaptation to the environment, 8) although learning is important in shaping our minds and behavior, its role is secondary and supplementary compared to the much larger role played by our genes in shaping our innate human nature.
The following quote from Steven Pinker (1997) expresses these ideas eloquently:
"The mind is a system of organs of computation, designed by natural selection to solve the kinds of problems our ancestors faced in their foraging way of life, in particular, understanding and outmaneuvering objects, animals, plants, and other people. [This view] can be unpacked into several claims. The mind is what the brain does; specifically, the brain processes information, and thinking is a kind of computation. The mind is organized into modules or mental organs, each with a specialized design that makes it an expert in one arena of interaction with the world. The modules' basic logic is specified by our genetic program. Their operation was shaped by natural selection to solve the problems of the hunting and gathering life led by our ancestors in most of our evolutionary history. The various problems for our ancestors were sub-tasks of one big problem for their genes, maximizing the number of copies that made it into the next generation."
How Genes Affect Body and Brain
Genes are responsible for producing the proteins that run everything in our bodies. Some proteins are visible, such as the ones that compose our hair and skin. Others work out of sight, coordinating our basic biological functions.
For the most part, every cell in our body contains exactly the same genes, but inside individual cells some genes are active while others are not. When genes are active, they are capable of producing proteins. This process is called gene expression. When genes are inactive, they are silent or inaccessible for protein production.
At least a third of the approximately 20,000 different genes that make up the human genome are active (expressed) primarily in the brain. This is the highest proportion of genes expressed in any part of the body. These genes influence the development and function of the brain, and ultimately control how we move, think, feel, and behave.
From DNA
In order to understand how genes work in the brain, we have to understand how genes make proteins. This begins with DNA (deoxyribonucleic acid).
DNA is a long molecule packaged into structures called chromosomes. Humans have 23 pairs of chromosomes, including a single pair of sex chromosomes (XX in females and XY in males). Within each pair, one chromosome comes from an individual’s mother and the other comes from the father. In other words, we inherit half of our DNA from each of our parents.
DNA consists of two strands wound together to form a double helix. Within each strand, chemicals called nucleotides are used as a code for making proteins. DNA contains only four nucleotides – adenine (A), thymine (T), cytosine (C), and guanine (G) – but this simple genetic alphabet is the starting point for making all of the proteins in the human body, estimated to be as many as one million. Coding this many proteins with just 4 "letters" is possible because these "letters" can be combined into long chains in an enormous number of different combinations and sequences.
To Gene
A gene is a stretch of DNA that contains the instructions for making or regulating a specific protein.
Genes that make proteins are called protein-coding genes. In order to make a protein, a molecule closely related to DNA called ribonucleic acid (RNA) first copies the code within DNA. Then, protein-manufacturing machinery within the cell scans the RNA, reading the nucleotides in groups of three. These triplets encode 20 distinct amino acids, which are the building blocks for proteins. The largest known human protein is a muscle protein called titin, which consists of about 27,000 amino acids.
Some genes encode small bits of RNA that are not used to make proteins, but are instead used to tell proteins what to do and where to go. These are called non-coding or RNA genes. There are many more RNA genes than protein-coding genes.
To Protein
Proteins form the internal machinery within brain cells and the connective tissue between brain cells. They also control the chemical reactions that allow brain cells to communicate with each other.
Some genes make proteins that are important for the early development and growth of the infant brain. For example, the ASPM gene makes a protein that is needed for producing new nerve cells (or neurons) in the developing brain. Alterations in this gene can cause microcephaly, a condition in which the brain fails to grow to its normal size.
Certain genes make proteins that in turn make neurotransmitters, which are chemicals that transmit information from one neuron to the next. Other proteins are important for establishing physical connections that link various neurons together in networks.
Still other genes make proteins that act as housekeepers in the brain, keeping neurons and their networks in good working order. Harmful mutations in these genes can cause some neurological diseases such as amytrophic lateral sclerosis (ALS, Lou Gehrig's Disease).
How Gene Expression Is Regulated
We know which protein a gene will make by looking at its code, also called its DNA sequence. What we cannot predict is the amount of protein that will be made, when it will be made, or what cell will make it.
Each cell turns on only a fraction of its genes, while it silences the rest. For example, genes that are expressed in brain cells may be silenced in liver cells or heart cells. Some genes are only turned on during the early months of human development and then are silenced later.
What determines these unique patterns of gene expression? Like people, cells have a unique lineage, and they tend to inherit traits from their parents. So, a cell’s origins influence the genes it turns on to make proteins. The cell’s environment – its exposure to surrounding cells and to hormones and other signals – also helps determine which proteins the cell makes. These cues from a cell’s past and from its environment act through many regulatory factors inside the cell, some of which are described in the following sections.
DNA Binding Proteins
About 10 percent of the genes in the human genome encode DNA binding proteins. Some of these proteins recognize and attach to specific bits of DNA to activate gene expression. Another type of DNA binding protein, called a histone, acts as a spool that can keep DNA in tight coils and thus suppress gene expression.
sRNA
Scattered throughout the genome are many types of small RNA (sRNA) that actively regulate gene expression. Because of their short length, they are able to target, match, and deactivate small bits of genetic code.
Epigenetic Factors
The word epigenetics comes from the Greek word epi, meaning above or beside. In a broad sense, epigenetics refers to long-lasting changes in gene expression without any changes to the genetic code. Epigenetic factors include chemical marks or tags on DNA or on histones that can affect gene expression. We shall discuss more about epigenetics later in this chapter.
Variations In Genetic Code
A genetic variation is a permanent change in the DNA sequence that makes up a gene. Most variations are harmless or have no effect at all. However, other variations can have harmful effects leading to disease. Still others can be beneficial in the long run, helping a species adapt to change.
Single Nucleotide Polymorphism (SNP)
SNPs are variations that involve a change in just one nucleotide. It is estimated that the human genome contains more than 10 million different SNPs. Because SNPs are such small changes within DNA, most of them have no effect upon gene expression. Some SNPs, however, are responsible for giving us unique traits, such as our hair and eye color. Other SNPs may have subtle effects on our risk of developing common diseases, such as heart disease, diabetes, or stroke.
Copy Number Variation (CNV)
At least 10 percent of the human genome is made up of CNVs, which are large chunks of DNA that are deleted, copied, flipped or otherwise rearranged in combinations that can be unique for each individual. These chunks of DNA often involve protein-coding genes. This means that CNVs are likely to change how a gene makes its protein.
Since genes usually occur in two copies, one inherited from each parent, a CNV that involves a single missing gene could lower the production of a protein below the amount needed.
Having too many copies of a gene can be harmful, too. Although most cases of Parkinson’s disease are sporadic (without a known cause), some cases have been linked to having two or more copies of the SNCA gene, which encodes a protein called alpha-synuclein. The excess alpha-synuclein accumulates in clumps inside brain cells, and appears to jam the cells’ machinery. For reasons that are not clear, similar clumps are associated with sporadic Parkinson’s disease.
Single Gene Mutation
Some genetic variations are small and affect only a single gene. These single gene mutations can have large consequences, however, because they affect a gene’s instructions for making a protein. Single gene mutations are responsible for many rare inherited neurological diseases, such as Huntington’s disease.
Behavioral Genetics
Behavioral genetics studies heritability of behavioral traits, and it overlaps with genetics, psychology, and ethology (the scientific study of human and animal behavior). Genetics plays a large role in when and how learning, growing, and development occurs. For example, although environment has an effect on the walking behavior of infants and toddlers, children are unable to walk at all before an age that is predetermined by their genome. However, while the genetic makeup of a child determines the age range for when he or she will begin walking, environmental influences determine how early or late within that range the event will actually occur.
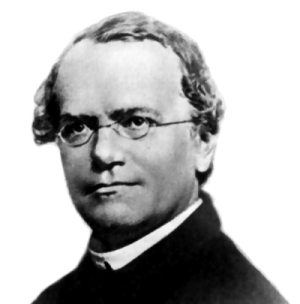
Classical Genetics
Classical, or Mendelian, genetics examines how genes are passed from one generation to the next, as well as how the presence or absence of a gene can be determined via sexual reproduction. Gregor Mendel is known as the father of the field of genetics, and his work with plant hybridization (specifically pea plants) demonstrated that certain traits follow particular patterns. This is referred to as the law of Mendelian inheritance.
Genes can be manipulated by selective breeding, which can have an enormous impact on behavior. For example, some dogs are bred specifically to be obedient, like golden retrievers; others are bred to be protective, like German shepards. In another example, Seymour Benzer discovered he could breed certain fruit flies with others to create distinct behavioral characteristics and change their circadian rhythms.
The Influence of Behavior on Genes
Behavior can influence genetic expression in humans and animals by activating or deactivating genes. Behavior can have an impact on genetic makeup, even as early as the prenatal period. It is important to understand the implications of behavior on genetic makeup in order to reduce negative environmental and behavioral influences on genes.
EEG and PET scans have the ability to show psychologists how certain behaviors trigger reactions in the brain. This has led to the discovery of specific genes, such as those that influence addictive behaviors. A variety of behaviors have been shown to influence gene expression, including—but not limited to—drug use, exposure to the elements, and dietary habits.
Drugs and Alcohol
Prenatal exposure to certain substances, particularly drugs and alcohol, has detrimental effects on a growing fetus. The most serious consequences of prenatal drug or alcohol exposure involve newborn addiction and fetal alcohol syndrome (FAS). Fetal alcohol syndrome affects both physical and mental development, damaging neurons within the brain and often leading to cognitive impairment and below-average weight. Exposure to drugs and alcohol can also influence the genes of children and adults. Addiction is thought to have a genetic component, which may or may not be caused by a genetic mutation resulting from drug or alcohol use.
Temperature
Temperature exposure can affect gene expression. For example, in Himalayan rabbits, the genetic expressions of fur, skin, and eyes are regulated by temperature. In the warm areas of the rabbits’ bodies, the fur lacks pigment due to gene inactivity and turns white. On the extremities of the rabbits’ bodies (nose, ears and feet) the gene is activated and therefore pigmented (usually black).
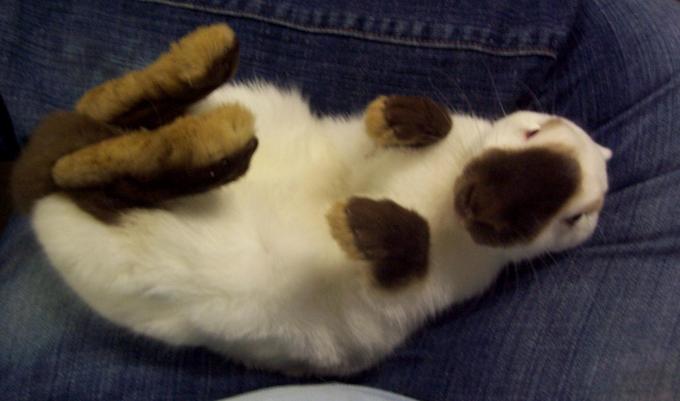
Light
Light exposure also influences genetic expression. Thomas Hunt Morgan performed an experiment in which he exposed some caterpillars to light and kept others in darkness. Those exposed to certain light frequencies had corresponding wing colors when they became butterflies (for example, red produced vibrant wing color, whereas blue led to pale wings). Darkness resulted in the palest wing color, leading him to conclude that light exposure influenced the genes of the butterflies. In this manner a caterpillar’s behavior can directly affect gene expression; a caterpillar that actively seeks out light will appear different as a butterfly than one that avoids it.
Nutrition
Lack of proper nutrition in early childhood is yet another factor that can lead to the alteration of genetic makeup. Human children who lack proper nutrition in the first three years of life tend to have more genetic problems later in life, such as health issues and problems with school performance.
Gene-Environment Interactions
Genes do not exist in a vacuum. Although we are all biological organisms, we also exist in an environment that is incredibly important in determining not only when and how our genes express themselves, but also in what combination. Each of us represents a unique interaction between our genetic makeup and our environment; range of reaction is one way to describe this interaction. Range of reaction asserts that our genes set the boundaries within which we can operate, and our environment interacts with the genes to determine where in that range we will fall. For example, if an individual’s genetic makeup predisposes her to high levels of intellectual potential and she is reared in a rich, stimulating environment, then she will be more likely to achieve her full potential than if she were raised under conditions of significant deprivation. According to the concept of range of reaction, genes set definite limits on potential, and environment determines how much of that potential is achieved. Some disagree with this theory and argue that genes do not set a limit on a person’s potential.
Another perspective on the interaction between genes and the environment is the concept of genetic environmental correlation. Stated simply, our genes influence our environment, and our environment influences the expression of our genes. Not only do our genes and environment interact, as in range of reaction, but they also influence one another bidirectionally. For example, the child of an NBA player would probably be exposed to basketball from an early age. Such exposure might allow the child to realize his or her full genetic, athletic potential. Thus, the parents’ genes, which the child shares, influence the child’s environment, and that environment, in turn, is well suited to support the child’s genetic potential.
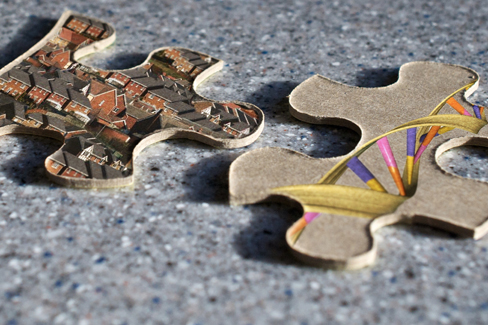
In another approach to gene-environment interactions, the field of epigenetics looks beyond the genotype itself and studies how the same genotype can be expressed in different ways. In other words, researchers study how the same genotype can lead to very different phenotypes. As mentioned earlier, gene expression is often influenced by environmental context in ways that are not entirely obvious. For instance, identical twins share the same genetic information (identical twins develop from a single fertilized egg that split, so the genetic material is exactly the same in each; in contrast, fraternal twins develop from two different eggs fertilized by different sperm, so the genetic material varies as with non-twin siblings). But even with identical genes, there remains an incredible amount of variability in how gene expression can unfold over the course of each twin’s life. Sometimes, one twin will develop a disease and the other will not. In one example, Tiffany, an identical twin, died from cancer at age 7, but her twin, now 19 years old, has never had cancer. Although these individuals share an identical genotype, their phenotypes differ as a result of how that genetic information is expressed over time. The epigenetic perspective is very different from range of reaction, because here the genotype is not fixed and limited.
Genes affect more than our physical characteristics. Indeed, scientists have found genetic linkages to a number of behavioral characteristics, ranging from basic personality traits to sexual orientation to spirituality (for examples, see Mustanski et al., 2005; Comings, Gonzales, Saucier, Johnson, & MacMurray, 2000). Genes are also associated with temperament and a number of psychological disorders, such as depression and schizophrenia. So while it is true that genes provide the biological blueprints for our cells, tissues, organs, and body, they also have significant impact on our experiences and our behaviors.
Let’s look at the following findings regarding schizophrenia in light of our three views of gene-environment interactions. Which view do you think best explains this evidence?
In a study of people who were given up for adoption, adoptees whose biological mothers had schizophrenia and who had been raised in a disturbed family environment were much more likely to develop schizophrenia or another psychotic disorder than were any of the other groups in the study:
- Of adoptees whose biological mothers had schizophrenia (high genetic risk) and who were raised in disturbed family environments, 36.8% were likely to develop schizophrenia.
- Of adoptees whose biological mothers had schizophrenia (high genetic risk) and who were raised in healthy family environments, 5.8% were likely to develop schizophrenia.
- Of adoptees with a low genetic risk (whose mothers did not have schizophrenia) and who were raised in disturbed family environments, 5.3% were likely to develop schizophrenia.
- Of adoptees with a low genetic risk (whose mothers did not have schizophrenia) and who were raised in healthy family environments, 4.8% were likely to develop schizophrenia (Tienari et al., 2004).
The study shows that adoptees with high genetic risk were especially likely to develop schizophrenia only if they were raised in disturbed home environments. This research lends credibility to the notion that both genetic vulnerability and environmental stress are necessary for schizophrenia to develop, and that genes alone do not tell the full tale.
Summary
Genes are sequences of DNA that code for a particular trait. Different versions of a gene are called alleles—sometimes alleles can be classified as dominant or recessive. A dominant allele always results in the dominant phenotype. In order to exhibit a recessive phenotype, an individual must be homozygous for the recessive allele. Genes affect both physical and psychological characteristics. The mind has evolved as a bundle of information processing modules "designed" by natural selection to increase survival and reproduction. Like other biological traits, the computational modules of the brain/mind are organized by genetic information during brain development. Ultimately, how and when a gene is expressed, and what the outcome will be—in terms of both physical and psychological characteristics—is a function of the interaction between our genes and our environments.
KEY TAKEAWAYS
Key Points
- Classical, or Mendelian, genetics examines how genes are passed from one generation to the next.
- Behavioral genetics examines the role of genetic and environmental influences on animal (including human) behavior.
- There are many ways to manipulate genetic makeup, such as cross-breeding to achieve certain characteristics.
- It is difficult to ascertain whether genetics (“nature”) or the environment (“nurture”) has a stronger influence on behavior. It is generally believed that human behavior is determined by complex interactions of both nature and nurture.
- Drug use, environmental exposure, and eating habits have all been linked to changes in gene expression. While some such influences are harmless or even beneficial, others can be extremely detrimental. Researchers hope to identify these behaviors and their effects.
- EEG and PET scans show psychologists how certain behaviors trigger reactions in the brain, which can lead to the discovery of certain determinant genes, such as those that influence addictive behaviors.
- Exposure of a fetus to alcohol and drugs can lead to a host of developmental problems after birth, the most serious of which is fetal alcohol syndrome.
Key Terms
- behavioral genetics: The field of study that examines the role of genetics in animal (including human) behavior; often involves the nature-versus-nurture debate.
- ethology: The scientific study of human and animal behavior.
- genetics: The branch of biology that deals with the transmission and variation of inherited characteristics, particularly chromosomes and DNA.
- gene: A unit of heredity; a segment of DNA or RNA that is transmitted from one generation to the next and that carries genetic information such as the sequence of amino acids for a protein.
- fetal alcohol syndrome: Any of a spectrum of birth defects resulting from excessive alcohol consumption by the mother during pregnancy.
Review Questions
A(n) ________ is a sudden, permanent change in a sequence of DNA.
- allele
- chromosome
- epigenetic
- mutation
________ refers to a person’s genetic makeup, while ________ refers to a person’s physical characteristics.
- Phenotype; genotype
- Genotype; phenotype
- DNA; gene
- Gene; DNA
________ is the field of study that focuses on genes and their expression.
- Social psychology
- Evolutionary psychology
- Epigenetics
- Behavioral neuroscience
Humans have ________ pairs of chromosomes.
- 15
- 23
- 46
- 78
Critical Thinking Questions
The theory of evolution by natural selection requires variability of a given trait. Why is variability necessary and where does it come from?
Personal Application Questions
You share half of your genetic makeup with each of your parents, but you are no doubt very different from both of them. Spend a few minutes jotting down the similarities and differences between you and your parents. How do you think your unique environment and experiences have contributed to some of the differences you see?
References
Cosmides, L., & Tooby, J. (1997). Evolutionary psychology: A primer. Center for Evolutionary Psychology. University of California, Santa Barbara.
Pinker, S. (1997). How the Mind Works. Norton. New York.
Attributions
"An Evolutionary and Computational View of the Mind" is original material written by Kenneth A. Koenigshofer, PhD., Chaffey College.
The remainder of this section is adapted by Kenneth A. Koenigshofer, Ph.D. from:
"How Genes affect Body and Brain" adapted from "Brain Basics: Genes at Work in the Brain," by National Institute of Neurological Disorders and Stroke (NINDS), https://www.ninds.nih.gov/Disorders/...nes-Work-Brain, All NINDS-prepared information is in the public domain and may be freely copied.
"Genetics and Behavior" by Boundless.com. License: CC BY-SA: Attribution-ShareAlike
From Openstax Psychology 2e Human Genetics License: Creative Commons Attribution Non-Commercial