6.2: Mechanisms of Psychoactive Drug Action
- Page ID
- 110457
This page is a draft and under active development. Please forward any questions, comments, and/or feedback to the ASCCC OERI (oeri@asccc.org).
- Describe the key elements of pharmacokinetics and their relationship to drug action.
- Compare and contrast the various drug administration methods in terms of potency, latency of action, and abuse potential.
- Describe the key elements of pharmacodynamics, particularly drug effects on steps of synaptic transmission and major neural circuitry
- Differentiate the major structures of the mesolimbic, "reward" pathway and the role of dopamine in its function.
Overview
Do drugs affect the body or does the body affect drugs? This may seem like either a very easy or a very odd question. Of course, the answer is drugs affect the body, right? For example, you take a certain drug and then your heart rate changes or you feel energized to move around a lot. In terms of psychoactive drugs, the brain, which is part of the body, is definitely affected by drugs. As we will see, neurotransmitters can be become more active and the receptors that these neurotransmitters bind to may trigger either increased or decreased communication with other neurons, glands, or muscles. These examples should make it clear that drugs definitely affect the body. However, even before these effects on the body occur, the body is able to affect incoming drugs in profound ways. Thus, the answer to the earlier question is that the body affects drugs and drugs affect the body.
This section will review the basics on how both of these processes occur with a general review of the concepts of pharmacokinetics (how the body affects drugs) and pharmacodynamics (how drugs affect the body, or more specifically the brain). In covering these topics we will address various physiological processes, such as drug metabolism and elimination and the influence of drug administration methods on a drug's impact. In addition, we will go into the specifics of how drugs interact with the process of synaptic communication and then zoom out to see how they alter the activity of major circuits and structures of the brain, such as the so-called, "reward pathway."
Pharmacokinetics: What Is It – Why Is It Important?
Pharmacokinetics refers to how the body processes drugs as they enter the body. While this section may sound more like pharmacology, it is important to realize how important pharmacokinetics can be when considering the ultimate effects of psychoactive drugs. As mentioned previously, psychoactive drugs exert their effects on behavior by altering neuronal communication in the brain, and the majority of drugs reach the brain by traveling in the blood.
The acronym ADME is often used to specify the processes of:
- A - Administration and Absorption (how the drug gets into the blood),
- D - Distribution (how the drug gets to the organ of interest – in this case, the brain),
- M - Metabolism (how the drug is broken down so it no longer exerts its psychoactive effects), and
- E - Elimination (how the drug leaves the body).
We will focus on some of these processes to show their importance in determining the effects of psychoactive drugs.
Drug Administration and Absorption
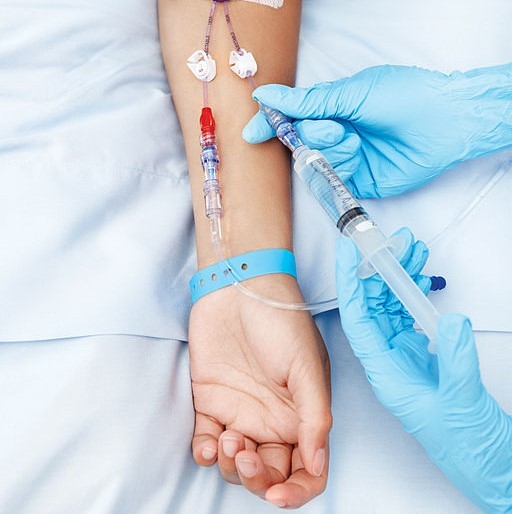
Before a drug can be absorbed by the body it needs to be administered in some way (i.e., the drug has to get into the body). There are many ways to take drugs, and these routes of drug administration can have a significant impact on how quickly that drug reaches brain.
The most common route of administration is oral administration, which begins in the mouth and continues in the digestive system. This route is relatively slow and – perhaps surprisingly – often the most variable and complex route of administration. Drugs enter the stomach and then get absorbed by the blood supply and capillaries that line the small intestine. The rate of absorption can be affected by a variety of factors including the quantity and the type of food in the stomach (e.g., fats vs. proteins). This is why the medicine label for some drugs (like antibiotics) may specifically state foods that you should or should not consume within an hour of taking the drug because they can affect the rate of absorption.
Two of the most rapid routes of administration include inhalation (i.e., smoking or gaseous anesthesia) and intravenous (IV) in which the drug is injected directly into the vein and hence the blood supply. Both of these routes of administration can get the drug to brain in less than 10 seconds. IV administration also has the distinction of being the most dangerous because if there is an adverse drug reaction, there is very little time to administer any antidote, as in the case of an IV heroin overdose.
Route of Administration | Typical Methods Used | Speed of Absorption in Blood | Advantages / Disadvantages |
---|---|---|---|
Oral (PO) | Pill, Liquid, Edibles | SLOWEST | Convenient, Gradual onset, Relatively safe / Variable blood levels due to individual differences in absorption and metabolism |
Subcutaneous | Injection into skin | ![]() |
Gradual onset, Avoids 1st pass metabolism / Only small volumes can be given, Possible localized irritation, tissue damage, infection |
Intramuscular (IM) | Injection into muscle | ![]() |
Steady absorption rates, Avoids 1st pass metabolism, Extended release possible / Only small volumes can be given, Possible localized irritation, tissue damage, infection |
Rectal | Rectal Suppository | ![]() |
Useful in those with digestive issues or unconscious, Mostly avoids 1st pass metabolism, Relatively high blood levels / Invasive, Unpredictable absorption rates |
Sublingual | Under tongue | ![]() |
Direct & fast absorption, Avoids 1st pass metabolism, Quick termination possible |
Inhalation | Smoke, Vape, Huff, Inhaler, Intranasal | ![]() |
Rapid absorption, Useful for emergency applications or localized effect (i.e. asthma), Reaches brain quickly / Variable dose & absorption, Relatively high abuse potential w/ certain drugs |
Intravenous | Injected into vein | FASTEST | Very rapid absorption (~instantly), Avoids 1st pass metabolism, Useful for emergency applications, Accurate dosing / High abuse potential and risk for overdose with certain drugs |
Why might knowing how quickly a drug gets to the brain be important? If we are considering therapeutic drugs, such as those used to treat anxiety, depression, or psychotic disorders, the time it takes to begin to relieve symptoms and how long this lasts can be critical information. For example, if treating an acute panic attack you would want a drug to act fast, whereas, if you want to prevent non-specific anxiety from occurring on a daily basis, you may use a different drug that acts more gradually but sticks around longer.
When considering potential drugs of abuse, administration and absorption rates are important for other reasons. Although there are multiple non-pharmacological risk factors for drug craving and substance use disorders, the phamacokinetics of a drug can play a significant role. For example, if a drug activates the reward circuits in the brain and reaches these areas quickly, the drug may have a relatively high risk for abuse and psychological dependence. Psychostimulants like amphetamine or cocaine are examples of drugs that have high risk for abuse in part because they are agonists at dopamine neurons involved in reward and because these drugs exist in forms that can be either smoked or injected intravenously. Some argue that cigarette smoking is one of the hardest addictions to quit, and although part of the reason for this may be that smoking gets the nicotine into the brain very quickly (and indirectly acts on dopamine neurons), it is a more complicated story.
For drugs that reach the brain very quickly, not only is the drug potentially very addictive, but so are the cues associated with the drug (see Rohsenow, Niaura, Childress, Abrams, and Monti, 1990). A learned connection between drug use and the environmental elements that accompany it can complicate quitting by triggering symptoms of withdrawal and/or cravings. For an IV opioid user, this could be the sight of a container in which the drug and syringes are typically stored or other aspects of their typical drug-taking environment. For a cigarette smoker, it could be the smell of another person's smoke or even something as typical as finishing dinner or waking up in the morning (if that is when the smoker usually has a cigarette). For both the opioid user and the cigarette smoker, the cues associated with the drug may actually cause craving that is alleviated by (you guessed it) – lighting a cigarette or injecting the opioid (i.e., relapse). This is one of the reasons individuals that enroll in drug treatment programs, especially out-of-town programs, are at significant risk of relapse if they later find themselves in proximity to old "environments", including friends and situations associated with drug use. But this is much more difficult for a cigarette smoker. How can someone avoid eating? Or avoid waking up in the morning, etc. These examples help you begin to understand how important the route of administration can be for psychoactive drugs.
Drug Metabolism
Metabolism involves the breakdown of psychoactive drugs, and this occurs primarily in the liver. The liver produces enzymes (proteins that speed up a chemical reaction), and these enzymes help catalyze a chemical reaction that breaks down psychoactive drugs. Enzymes exist in “families,” and many psychoactive drugs are broken down by the same family of enzymes, the Cytochrome P450 super family. Usually, there is not a unique enzyme for each drug; rather, certain enzymes can break down a wide variety of drugs. Tolerance to the effects of many drugs can occur with repeated exposure; that is, the drug produces less of an effect over time, so more of the drug is needed to get the same effect. This is particularly true for sedative drugs like alcohol or opiate-based painkillers (e.g., fentanyl, codeine). Metabolic tolerance is one kind of tolerance and it takes place primarily in the liver. Some drugs, such as alcohol cause enzyme induction – an increase in the enzymes produced by the liver. For example, chronic drinking results in alcohol being broken down more quickly, so the alcoholic needs to drink more to get the same effect – of course, until so much alcohol is consumed that it damages the liver (i.e. fatty liver or cirrhosis).
There are a variety of other ways that enzyme action can be altered by both physiological and environmental aspects of drug taking. For example, just the expectation of drug taking or the cues from the environment where drug taking regularly occurs can trigger either enzyme induction or inhibition and, thus altering the ultimate physical and/or psychological effects of the drug. These differences in response can be particularly dangerous if they involve an unexpected increase in life-threatening side effects, such as the respiratory depression seen in response to opioids.
After "surviving" the processes of absorption and initial metabolism but before being eliminated, drugs are distributed throughout the body until they reach their target. In the case of psychoactive drugs, the main target is the brain. At this point, the process shifts to pharmacodynamics where the roles reverse and drugs are now able to affect the body.
Pharmacodynamics
If you have a headache or muscle pain, you might take a drug like ibuprofen (Advil, Motrin) or acetaminophen (Tylenol). Part of how these drugs work is to block the production of chemicals that cause the inflammation that is responsible for the pain. Thus, their target is the location of action is where the inflammation or pain is occurring. For psychoactive drugs, including those which treat pain the target of action for their effects is the brain or nervous system. The pharmacodynamics of their effects can be divided into two general categories: Their influence on specific steps of communication at the level of the synapse and the more holistic effect on the activity of certain circuits or major structures of the nervous system.
Just about all psychoactive drugs have their initial effects on one or more of the steps of synaptic communication: neurotransmitter synthesis, storage, release, binding, reuptake, and breakdown (See Figure 6.2.2). Remember, drugs can act as either agonists or antagonists of a neurotransmitter's typical effects. The drug L-dopa, for example acts as an agonist to increase the synthesis of dopamine and treat the dopamine dysfunction present in Parkinson's disease. Other agonist drugs like amphetamine and some other stimulants can increase the amount of dopamine activity by enhancing the release step. And yet others, such as cocaine can increase the synaptic activity of dopamine by blocking its reuptake. All these drugs are dopamine agonists (increase dopamine synaptic activity), but they can have very different psychological and behavioral effects due to the difference in the synaptic step targeted.
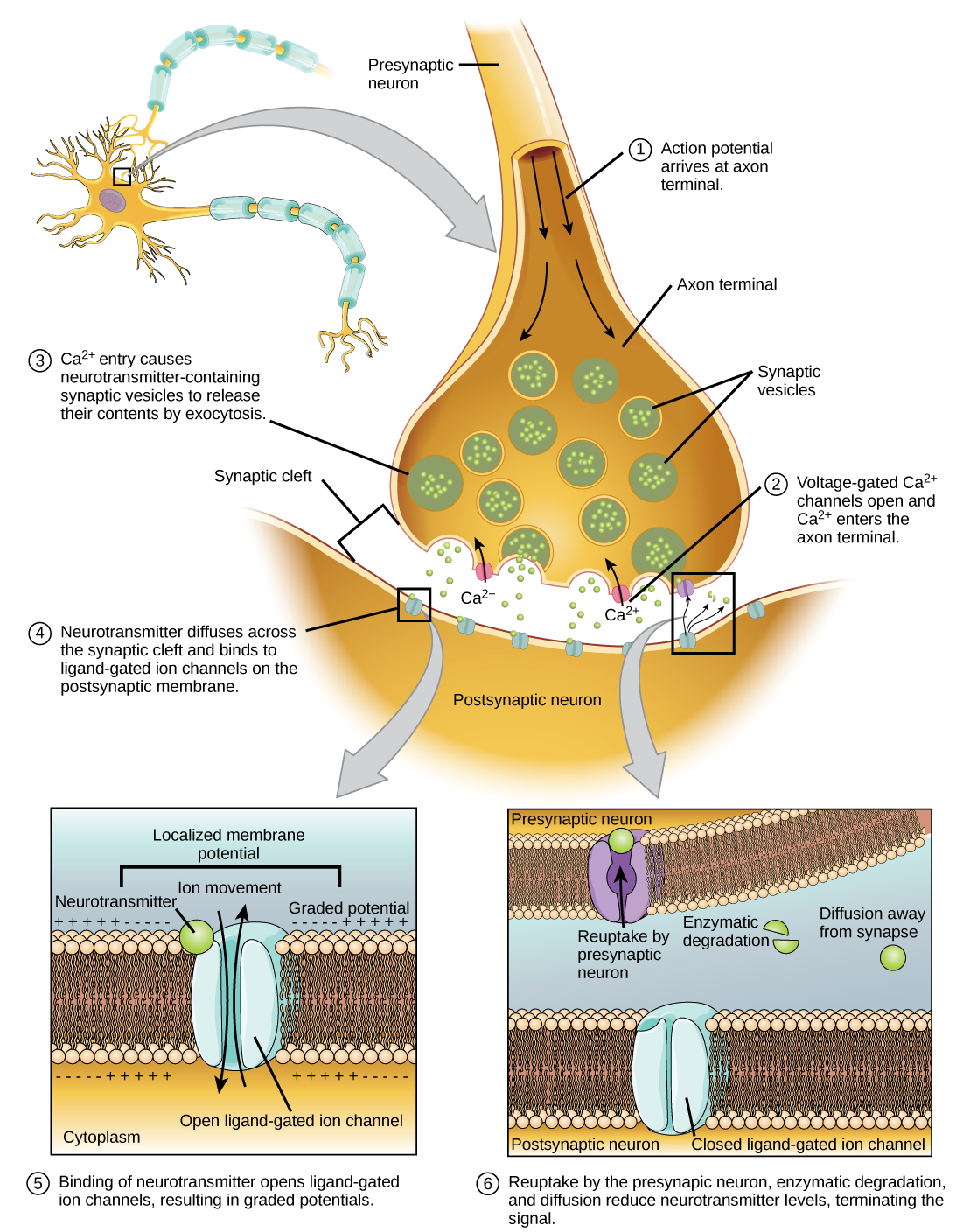
Knowing these initial actions of drugs at the level of the synapse is important in order to understand how they ultimately affect our thinking, feeling, and behavior. However, the thousands or even millions of synaptic changes that occur every split second due to drug action first need to be integrated in order to have noticeable effects. This integration is reflected in alterations of the activity of key structures and circuits of the nervous system.
Drug effects on Neural Structures and Circuits
Every distinct brain area or neural circuit can be affected in some way by the action of psychoactive drugs due to the integration process discussed above. For example, drugs that increase endorphin activity (i.e. Opioids) in certain areas of the brain can trigger changes in a major pain-inhibition pathway. Circuits involved with attention are affected by drugs that enhance norepinephrine systems and can be effective treatments for ADHD. Still other drugs, such as anti-anxiety medications (i.e. Xanax, Valium) are GABA agonists and can enhance the action of GABA throughout the brain, including the amygdala, which plays a significant role in fear and anxiety responses.
Mesolimbic "Reward" Circuitry
One of the most interesting and significant circuits that has been studied for its role in drug taking and substance use disorder is the "reward circuit" or "pleasure pathway" of the brain. This circuitry is technically called the mesolimbic circuitry and contains major dopamine pathways in addition to other transmitters which play a role in the positive feelings associated with both natural and drug rewards (See Figure 6.2.3).
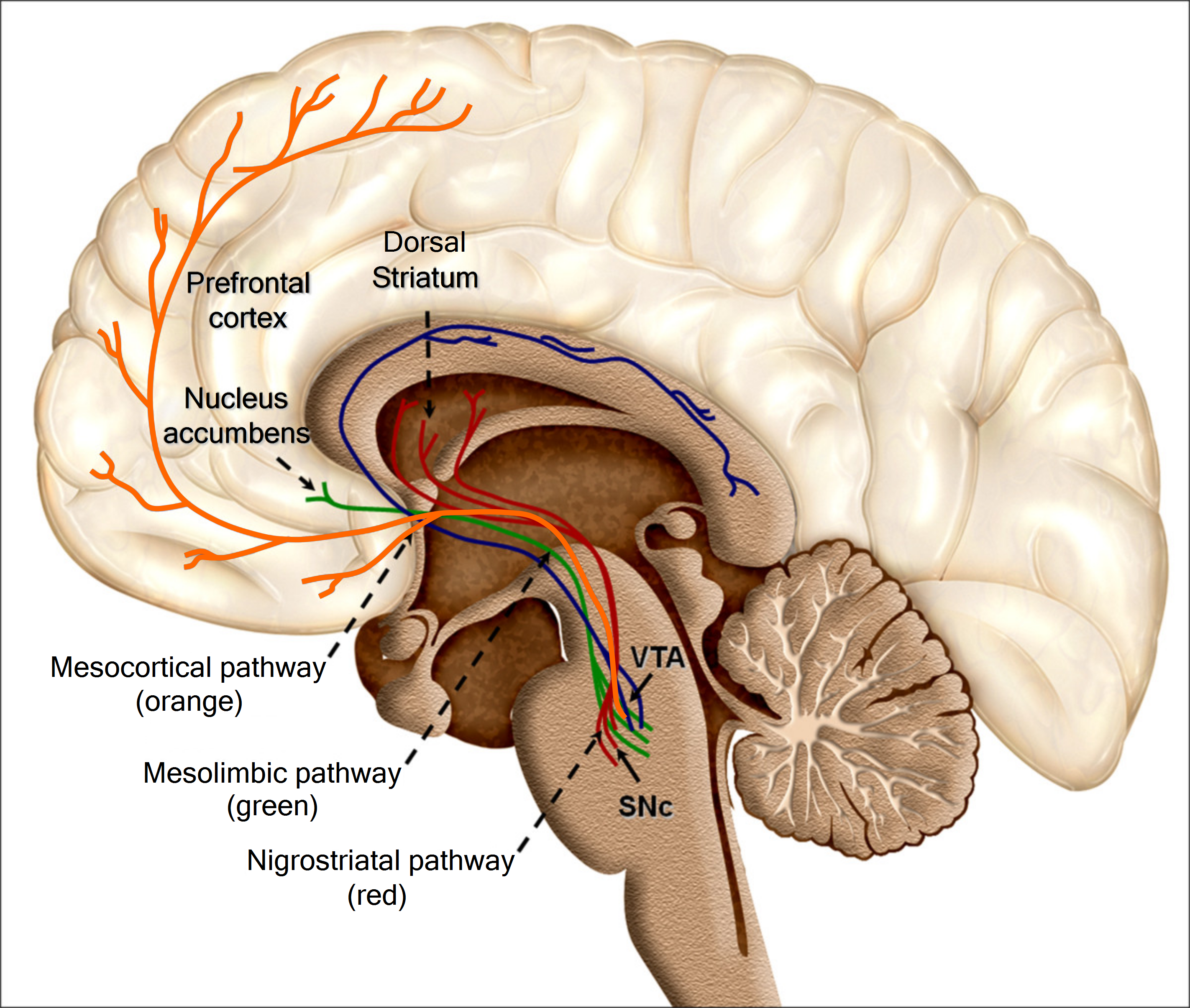
The nucleus accumbens definitely plays a central role in the reward circuit. Its operation is based chiefly on two essential neurotransmitters: dopamine, which promotes desire, and serotonin, whose effects include satiety and inhibition. Many animal studies have shown that many psychoactive drugs and natural rewards increase the production of dopamine in the nucleus accumbens, while reducing that of serotonin.
But the nucleus accumbens does not work in isolation. It maintains close relations with other areas involved in pleasure and reward. One area in particular is the ventral tegmental area (VTA). Located in the midbrain, at the top of the brainstem, the VTA is one of the most primitive parts of the brain. Neurons of the VTA synthesize dopamine and then send it via their axons primarily to the nucleus accumbens. The VTA is also influenced by endorphins whose receptors are targeted by opiate drugs such as heroin and morphine.
Another structure involved in pleasure mechanisms is the prefrontal cortex, whose role in planning and motivating action is well established. The prefrontal cortex is a significant relay in the reward circuit and also is modulated by dopamine.
The locus coeruleus, an alarm area of the brain and packed with norepinephrine, is another brain structure that plays an important role in drug abuse. When stimulated by a lack of the drug in question, the locus coeruleus drives the user to do anything necessary to obtain more drug.
Three other structures in the limbic system also play an active part in the pleasure circuit and, consequently, in drug dependency. The first is the amygdala, which can provide affective or mood information in response to drugs or environmental stimuli.
The second is the hippocampus, which preserves the agreeable memories associated with drug taking or other non-drug behaviors and, by association, all of the details of the environment in which these behaviors occur. The memory of these details may trigger positive feelings and, in the case of drugs reawaken the desire to take the drug again contributing to the possibility of relapse.
The third structure, the most anterior portion of the insular cortex, or insula, is regarded as part of the limbic system that is thought to play a role in the active pleasure-seeking associated both with food and with psychoactive substances. It has been proposed that this part of the cortex tells us about the bodily states associated with our emotional experiences and then relates these feeling states to cognitive processes such as decision-making (Damasio et al., 2013).
Summary
Psychoactive drugs clearly undergo quite a journey through the body and, ultimately the brain prior to causing changes in how we think, feel, perceive the world, and behave. The pharmacokinetic steps of administration, distribution, metabolism, and elimination have a major impact on the timing and amount of drug action prior to their arrival at their targets in the nervous system. Upon arrival, pharmacodynamic actions primarily at the synapse contribute to the diverse and dynamic action of drugs on neural communication and subsequent psychological and behavioral changes. Psychoactive drugs have the potential to affect structures in all parts of the nervous system as long as the appropriate receptors are there to receive the. The structures of the Mesolimbic Reward Circuitry play a particularly critical role in the action of many psychoactive drugs, both therapeutic and recreational.
Attributions
- Pharmacokinetics adapted from ""NOBA - Psychology - Section 9.12, Psychopharmacology." License: CC BY SA-NC 4.0 International
References
- Damasio A, Damasio H, Tranel D. (2013) Persistence of feelings and sentience after bilateral damage of the insula. Cereb Cortex. 23(4):833-46. doi: 10.1093/cercor/bhs077. PMID: 22473895.
- Rohsenow DJ, Niaura RS, Childress AR, Abrams DB, Monti PM. (1990-1991) Cue reactivity in addictive behaviors: theoretical and treatment implications. Int J Addict. 25(7A-8A):957-93. DOI: 10.3109/10826089109071030. PMID: 2131326.