12.1: Maintaining Water Balance
- Page ID
- 137596
This page is a draft and under active development. Please forward any questions, comments, and/or feedback to the ASCCC OERI (oeri@asccc.org).
- Discuss the importance of water balance.
- Explain the role of hormones in maintaining water balance.
- Describe the renin-angiotensin-aldoesterone system and how it impacts blood volume and pressure.
Overview
As with eating behavior, environmental factors also impact our intake of fluids. But while we have varied sources of energy and mechanisms for storing that energy, the need to drink is triggered by a disruption in water balance. What makes thirst and drinking more complicated is the fact that water imbalances can be created by either a loss of fluids or an increase in solutes that causes water to move out of calls. In other words, just as it appears that we monitor both short-term and long-term energy stores to trigger hunger, we also monitor two different water stores to determine a need to drink. We'll explore this in more detail following an overview of the biological controls of water balance and the brain mechanisms that trigger thirst.
Maintaining Water Balance - Physiological Mechanisms
Maintaining a proper water balance in the body is important to avoid dehydration or over-hydration. The water concentration of the body is monitored by osmoreceptors in the hypothalamus, which detect the concentration of electrolytes in the extracellular fluid. The concentration of electrolytes in the blood rises when there is water loss caused by excessive perspiration, inadequate water intake, or low blood volume due to blood loss. An increase in blood electrolyte levels results in a neuronal signal being sent from the osmoreceptors in hypothalamic nuclei.
The hypothalamus produces a polypeptide hormone known as antidiuretic hormone (ADH, also known as vasopressin), which is transported to and released from the posterior pituitary gland. The principal action of ADH is to regulate the amount of water excreted by the kidneys. As ADH causes direct water reabsorption from the kidney tubules, salts and wastes are concentrated in what will eventually be excreted as urine. The hypothalamus controls the mechanisms of ADH secretion, either by regulating blood volume or the concentration of water in the blood. Dehydration or physiological stress can cause an increase in solute concentration which leads to ADH secretion and the retention of water, causing an increase in blood pressure. ADH travels in the bloodstream to the kidneys. Once at the kidneys, ADH changes the kidneys to become more permeable to water by temporarily inserting water channels, aquaporins, into the kidney tubules. Water moves out of the kidney tubules through the aquaporins, reducing urine volume. The water is reabsorbed into the capillaries returning the blood's solute levels back toward normal. As the blood solute concentration decreases, a negative feedback mechanism reduces osmoreceptor activity in the hypothalamus, and ADH secretion is reduced. ADH release can be reduced by certain substances, including alcohol, which can cause increased urine production and dehydration.
Chronic underproduction of ADH or a mutation in the ADH receptor results in diabetes insipidus, a condition characterized by the rapid loss of water following intake. If the posterior pituitary does not release enough ADH, water cannot be retained by the kidneys and is lost as urine. This causes increased thirst, but water taken in is lost again and must be continually consumed. If the condition is not severe, dehydration may not occur, but severe cases can lead to electrolyte imbalances due to dehydration.
Another hormone responsible for maintaining electrolyte concentrations in extracellular fluids is aldosterone, a steroid hormone that is produced by the adrenal cortex. In contrast to ADH, which promotes the reabsorption of water to maintain proper water balance, aldosterone maintains proper water balance by enhancing sodium (Na+) reabsorption and potassium (K+) secretion from extracellular fluid of the cells in kidney tubules. Because it is produced in the cortex of the adrenal gland and affects the concentrations of minerals Na+ and K+, aldosterone is referred to as a mineralocorticoid, a corticosteroid that affects ion and water balance. Aldosterone release is stimulated by a decrease in blood sodium levels, blood volume, or blood pressure, or an increase in blood potassium levels. It also prevents the loss of Na+ from sweat, saliva, and gastric juice. The reabsorption of Na+ also results in the osmotic reabsorption of water, which alters blood volume and blood pressure.
Aldosterone production can be stimulated by low blood pressure, which triggers a sequence of chemical release, as illustrated in Figure \(\PageIndex{1}\). When blood pressure drops, the renin-angiotensin-aldosterone system (RAAS) is activated. Cells in the juxtaglomerular apparatus, which regulates the functions of the nephrons of the kidney, detect this and release renin. Renin, an enzyme, circulates in the blood and reacts with a plasma protein produced by the liver called angiotensinogen. When angiotensinogen is cleaved by renin, it produces angiotensin I, which is then converted into angiotensin II in the lungs. Angiotensin II functions as a hormone and then causes the release of the hormone aldosterone by the adrenal cortex, resulting in increased Na+ reabsorption, water retention, and an increase in blood pressure. Angiotensin II in addition to being a potent vasoconstrictor also causes an increase in ADH and increased thirst, both of which help to raise blood pressure.
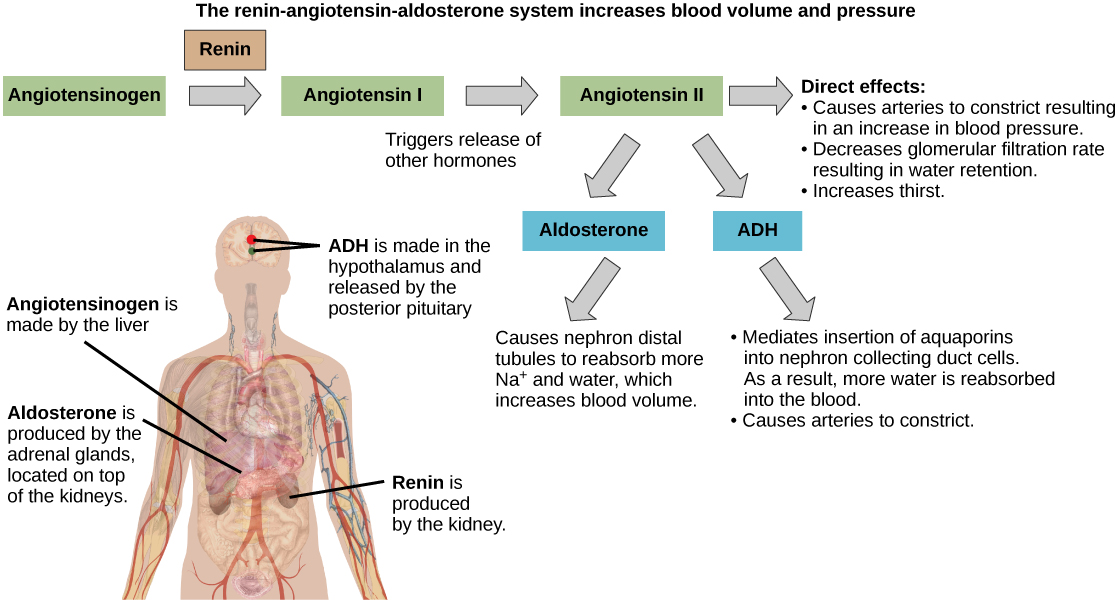
Maintaining Water Balance - Triggering Thirst
When your body starts to run low on water, a number of changes take place: for one, the volume of your blood decreases, causing a change in blood pressure. Because the amount of salt and other minerals in your body is staying constant as the volume of liquids decreases, their relative concentration increases (the same number of particles in a smaller volume means that the particles are more concentrated). This concentration of particles in bodily fluids relative to the total amount of liquid needs to be kept in a narrow range to keep the cells in your body functioning properly. Your body also needs a steady supply of fluids to transport nutrients, eliminate waste, and lubricate and cushion joints. To some extent, the body can compensate for water depletion by altering heart rate and blood pressure and by modifying kidney function to retain more water. For you, though, the most noticeable indication that your body is running low on fluids is likely the feeling of thirst, as you increasingly feel like you need to drink some water.
So how does your body know that these responses are necessary, and how are they coordinated across so many different organ systems? Research indicates that a highly specialized part of the brain called the lamina terminalis is responsible for guiding many of these thirst responses (Figure \(\PageIndex{2}\)). Brain cells within the lamina terminalis can sense when the body is running low on water and whether you’ve had anything to drink recently. When researchers manipulate this brain region, they can also drive animals to seek out or avoid water, regardless of how hydrated that animal might be.
The lamina terminalis is located towards the front of the brain and occupies a prime location just below a fluid reservoir called the third ventricle. Unlike much of the rest of the brain, many cells in the lamina terminalis aren’t guarded by a blood-brain barrier. This barrier prevents many circulating factors in the blood and other fluids from interacting with cells in the brain, offering the brain protection against potentially dangerous invaders like certain bacteria, viruses, and toxins. However, the blood-brain barrier also cuts the brain off from many circulating signals that might hold useful information about the body’s overall status. Because certain cells in the lamina terminalis lie outside the blood-brain barrier, these cells can also interact with the fluid in the third ventricle to keep tabs on factors that indicate whether the body needs more or less water. In particular, these cells can monitor the fluid in the ventricle to determine its osmolality (the ratio of salt particles to a given amount of liquid) and the amount of sodium present.
When other parts of the brain detect information that’s relevant to understanding the body’s water needs, they frequently pass it along to the lamina terminalis, as well (Figure \(\PageIndex{2}\)). In this way, the lamina terminalis also collects information about things like blood pressure, blood volume, and whether you’ve eaten recently (even before food can cause any change in circulating salt or water levels, your body tries to maintain a balance between these factors by encouraging you to drink water every time you eat). Information from the part of the brain that controls the circadian clock (the suprachiasmatic nucleus of the hypothalamus) also gets forwarded to the lamina terminalis, encouraging animals to drink more water before sleeping to avoid becoming dehydrated during long periods of sleep. Collectively, this information gives the lamina terminalis the resources needed to make a call about whether the body needs more or less water. In turn, cells in the lamina terminalis project to many other areas of the brain, sending out their verdict about current water needs. Although scientists are still trying to figure out exactly how information from the lamina terminalis affects other brain regions, it’s clear that this output can influence an animal’s motivation to seek out water, as well as physiological factors like kidney function and heart rate (Figure \(\PageIndex{3}\)).
What makes water so refreshing?
After a while standing outside in the hot sun, a cold drink of water tends to feel instantly refreshing. You might also find that drinking a very sugary beverage feels equally refreshing but leaves you feeling thirsty again later. In both cases, it takes tens of minutes for that drink to have any effect on attributes like osmolality or blood pressure, the body’s main indicators of hydration status. Instead, the brain must rely on some other cue to tell you to stop drinking and give you that instant feeling of refreshment.
The Role of the Lamina Terminalis
Researchers have discovered that neurons in the lamina terminalis respond to the physical act of swallowing liquids, even before there are any changes in the amount of water in the blood. Researchers have identified a group of neurons in the lamina terminalis whose activity is required for drinking behaviors: when you artificially turn off the activity in these cells, mice no longer drink water, even when they are water deprived (Zimmerman et al., 2017). When the researchers recorded the activity of these cells as animals drank water, they found that the cellular activity decreased in lockstep with each sip of water, far before any physiological changes in blood pressure or solute concentration could have an effect. They also found that this change in activity only happened when the mice drank water, not when they drank a salt solution. This study suggests that our brains have a built-in mechanism to compare how much water we need with the amount of water we’re currently drinking, telling us when we’ve had enough and leaving us feeling instantly hydrated. Still, scientists don’t know exactly how the brain can tell water apart from other liquids, or why drinking some non-water beverages can leave you feeling instantly hydrated, as well.
The Role of Dopamine
Another group of researchers set out to tackle the problem of why we find drinking water so rewarding when we’re thirsty (Augustine at al., 2019). Neuroscientists have long recognized the role of dopamine in determining what we find reinforcing. In order to look at the role that dopamine plays with respect to drinking behaviors, these researchers used a new kind of sensor that glows in the presence of dopamine. By putting this sensor into a mouse’s brain, they were able to record dopamine levels in real time as the mouse went about its tasks (Figure \(\PageIndex{4}\).
These researchers looked at dopamine levels after thirsty mice drank water and other liquids. They also recorded dopamine levels after they injected water directly into the gastrointestinal system; this procedure hydrated thirsty animals, but meant that the mice didn’t actually drink any water. Oka’s group found that thirsty mice had a large surge in dopamine levels after drinking either water or saline and that these dopamine changes happened even before drinking would have any effect on blood fluid levels. In contrast, the animals didn’t release any dopamine after water was pumped into their gastric systems, suggesting that it’s the act of drinking itself that’s rewarding—not the feeling of being hydrated. This effect also starts to explain why drinking beverages other than water can be so satisfying, even when they leave you feeling thirsty later: the dopamine spike that comes from drinking liquids when animals are thirsty doesn’t depend on what kind of liquid they’re drinking, even though not all liquids are equally hydrating.
These two studies highlight the varying strategies the brain uses to monitor essential nutrients like water; because no single sensor can tally current water levels and predict future water needs, the brain relies on a variety of sensations and cues.
Summary
XXXX
References
Augustine, V., Ebisu, H., Zhao, Y., Lee, S., Ho, B., Mizuno, G. O., Tian, L., & Oka, Y. (2019). Temporally and spatially distinct thirst satiation signals. Neuron, 103(2). https://doi.org/10.1016/j.neuron.2019.04.039
Zimmerman, C. A., Lin, Y. C., Leib, D. E., Guo, L., Huey, E. L., Daly, G. E., Chen, Y., & Knight, Z. A. (2016). Thirst neurons anticipate the homeostatic consequences of eating and drinking. Nature, 537(7622), 680–684. https://doi.org/10.1038/nature18950
Attributions
"Maintaining Water Balance - Physiological Mechanisms" adapted from Rye, C., Wise, R., Jurukovski, V., DeSaix, J., Choi, J., & Avissar , Y. (2016, October 21). 37.3 Regulation of Body Processes - Biology. OpenStax. Retrieved February 6, 2022, from https://openstax.org/books/biology/p...body-processes (CC BY)
"Maintaining Water Balance - Triggering Thirst" adapted from Frank, M. (2019, September 26). The Neuroscience of Thirst: How your brain tells you to look for water. Retrieved May 9, 2022 from https://sitn.hms.harvard.edu/flash/2...ls-look-water/ (CC BY-NC-SA)