3.5: Genomics, Epigenetics, and Genetic Testing
- Page ID
- 158733
GENOMICS
All the genetic information of an organism.
A molecular laboratory procedure that produces the order of nucleotide bases (i.e., sequences).
A molecular procedure that is performed to test for the presence of certain alleles or to discover new ones.
A genetic mutation (i.e., allele) that has a harmful phenotypic disease-causing effect.
EPIGENETICS
Methyl groups bind DNA, which modifies the transcriptional activity of a gene by turning it “on” or “off.”
The methylation pattern throughout a genome—that is, which genes (and other genomic sites) are methylated and unmethylated.
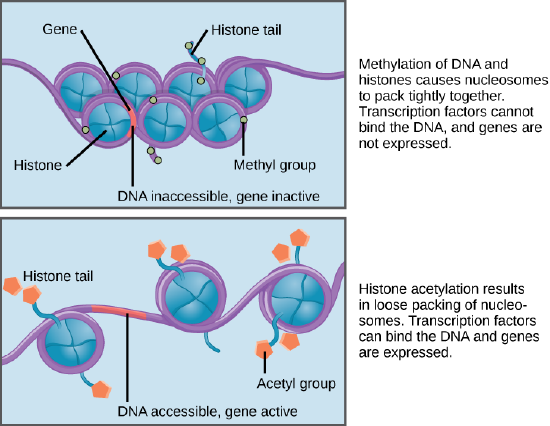
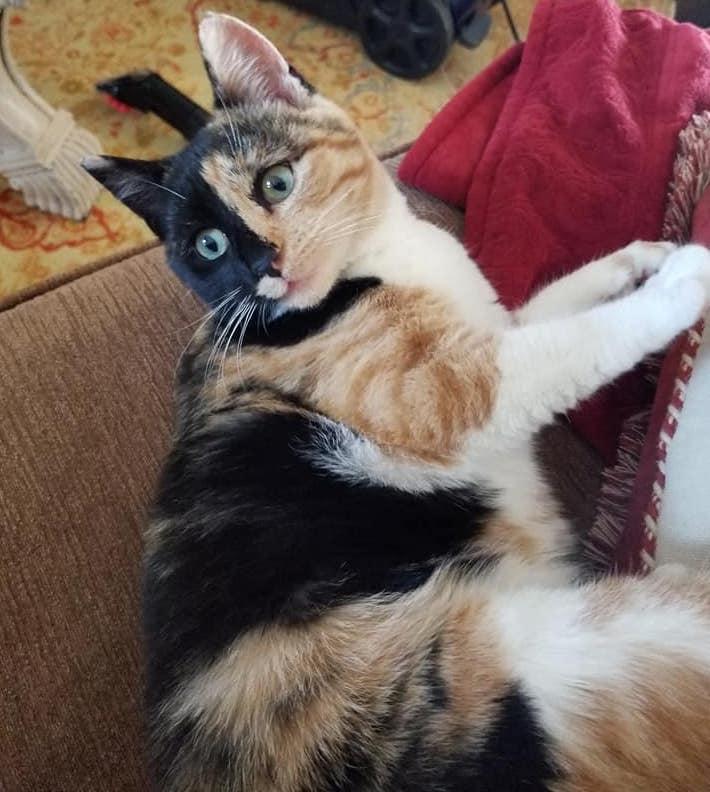
In order to assist with public health efforts, newborn screening for genetic diseases have been available in the United States for over 50 years. One of the first available genetic tests was to confirm a phenylketonuria (PKU) diagnosis in infants, which is easily treatable with a dietary change. Currently, each state decides what genes are included on newborn screening panels and some states even have programs to help with infant medical follow-ups.
There are now hundreds of laboratories that provide testing for a few thousand different genes that can inform medical decisions for infants and adults. What has made this industry possible are the advancements in technology and decreased cost to patients. Moreover, genetic testing has been made available publicly to anyone without the assistance of medical professionals.
GENETIC TESTING
Polymerase Chain Reaction (PCR) and Sanger Sequencing
One of the most important inventions in the genetics field was polymerase chain reaction (PCR). In order for researchers to visualize and therefore analyze DNA, the concentration must meet certain thresholds. In 1985, Kary Mullis developed PCR, which can amplify millions of copies of DNA from a very small amount of template DNA (Figure 3.40). For example, a trace amount of DNA at a crime scene can be amplified and tested for a DNA match. Also, aDNA is typically degraded, so a few remaining molecules of DNA can be amplified to reconstruct ancient genomes. The PCR assay uses similar biochemical reactions to our own cells during DNA replication.
A molecular biology procedure that can make copies of genomic DNA segments. A small amount of DNA is used as a starting template and is then used to make millions of copies.
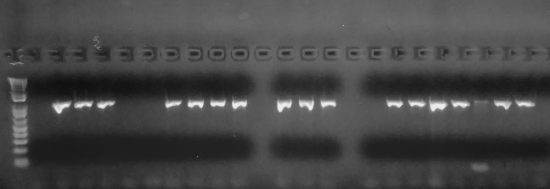
In Sanger sequencing, PCR sequences can be analyzed at the nucleotide level with the help of fluorescent labeling. Several different types of alleles and genetic changes can be detected in DNA by using this analysis. Figure 3.5.4 shows someone who is heterozygous for a single nucleotide allele. These methods continue to be used extensively alongside larger-scale genome technologies.
A process that involves the usage of fluorescently labeled nucleotides to visualize DNA (PCR fragments) at the nucleotide level.
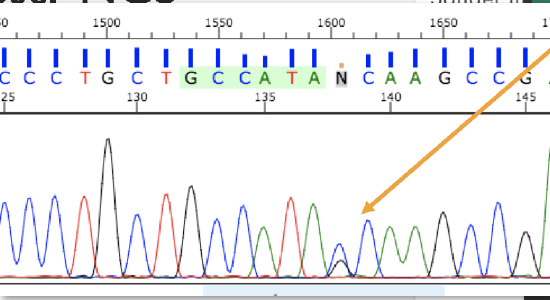
Genetic Biotechnology and Clinical Testing
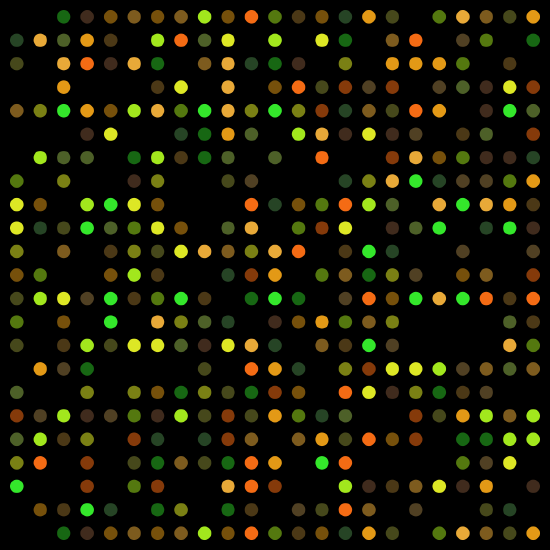
Genetic innovations are transforming the healthcare industry. However, the different types of technology and the results of these tests often include a learning curve for patients, the public, and medical practitioners. Microarray technology, when DNA samples are genotyped (or “screened”) for specific alleles, has been available for quite some time (Figure 3.42). Presently, microarray chips can include hundreds of alleles that are known to be associated with various diseases. The microarray chip only binds with a DNA sample if it is “positive” for that particular allele and a fluorescent signal is emitted, which can be further analyzed.
A genotyping procedure that utilizes a microarray chip, which is a collection of thousands of short nucleotide sequences attached to a solid surface that can probe genomic DNA.
If a patient is suspected of having a rare genetic condition that cannot be easily diagnosed or the diagnosis is entirely unknown, whole genome sequencing may be recommended by a doctor. Next-generation sequencing (NGS) is a newer technology that can screen the entire genome by analyzing millions of sequences within a single machine run (Figure 3.5.6). However, sequencing the entire genome yields a significant amount of data and information. Therefore, clinical NGS genetic testing typically only includes a small subset of the genome known to have pathogenic disease-causing mutations.
A genotyping technology that involves producing millions of nucleotide sequences (from a single DNA sample) that are then read with a sequencing machine. It can be used for analyzing entire genomes or specific regions and requires extensive program-based applications.
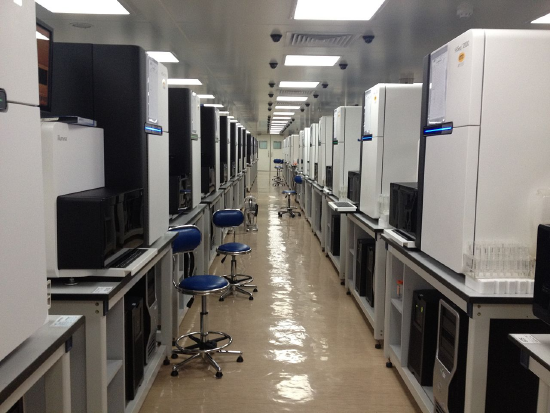
There is a diversity of clinical genetics tests available to assist patients with making medically informed decisions about family planning and health, including assistance with in vitro fertilization (IVF) procedures and embryo genetic screening. To ensure accuracy, it is highly important that all clinical laboratories are continually regulated. The Clinical Laboratory Improvement Amendments (CLIA) are United States federal standards that all human laboratory testing clinics must follow. A major benefit provided by some clinical genetic testing companies is access to genetic counselors, who have specialized education and training in medical genetics and counseling. Both partners are usually tested to see if there is a risk for passing on a disease to a child. Counselors use their skillset to aid patients and doctors with risk assessment for genetic diseases and interpretation of genetic testing results. Genetic counselors also guide and support patients when making impactful medical decisions.
Direct-to-Consumer (DTC) Genetic Testing
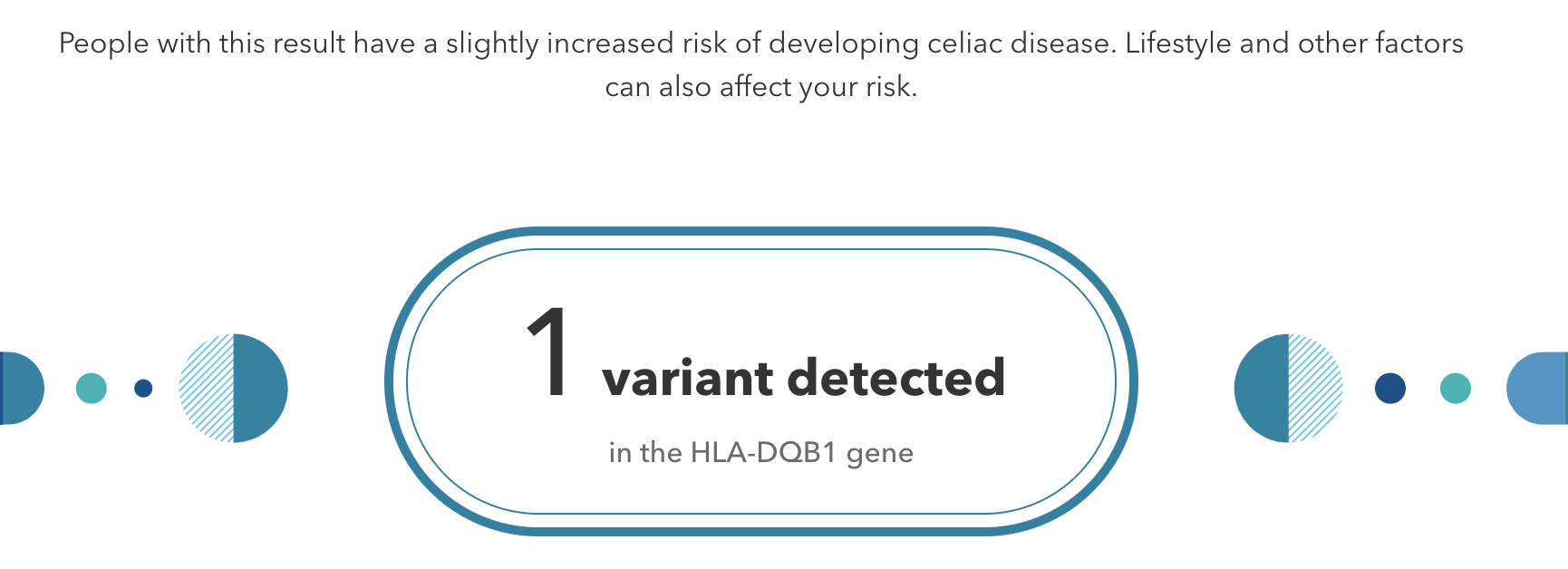
Genetic testing that is performed without the guidance of medical professionals is called direct-to-consumer (DTC) genetic testing. Companies that sell affordable genome sequencing products to the public continue to increase in number and popularity. These companies have marketing campaigns typically based on the notion of “personal empowerment,” which can be achieved by “knowing more about your DNA.” For example, if you are identified as having a slightly increased risk for developing celiac disease (Figure 3.5.7), then you may be motivated to modify your dietary consumption by removing gluten from your diet. Another scenario is that you could test positive for a known pathogenic BRCA1 or BRCA2 allele. In this case, you may want to follow up with additional testing from a medical facility, which could lead to life-altering decisions. DNA sequencing products for entertainment purposes are also available. For example, by having your “Vinome” analyzed, you can discover if you are more predisposed to liking Pinots as your favorite wine. Additionally, an individual and their partner can be genotyped to predict what physical characteristics their baby might inherit.
DTC testing typically lacks genetic counselor services and regulations are not as strict. This has led to some controversies including company genetic products that provide health information. The company 23andMe was the first on the market to offer DTC health testing, and in 2013, the U.S. Food and Drug Administration (FDA) intervened. 23andMe worked toward complying with FDA regulations and then gained approval to offer testing on a few medically related genes. In 2017, 23andMe offered a “Late-Onset Alzheimer’s Disease” genetic risk report. Such offerings have been criticized because customers could receive results they may not fully be able to interpret. In turn, this could increase the stress of participants (sometimes called the “burden of knowing”) and could also lead to unnecessary medical intervention. In order to address this issue, 23andMe now provides disclaimers and also interactive learning modules that customers must complete if they wish to view certain genotyping results. However, individuals who tested positive for a disease-causing allele have also been able to successfully seek medical help. The potential for harm and the proposed benefits of DTC testing continue to be a topic of debate and investigation.
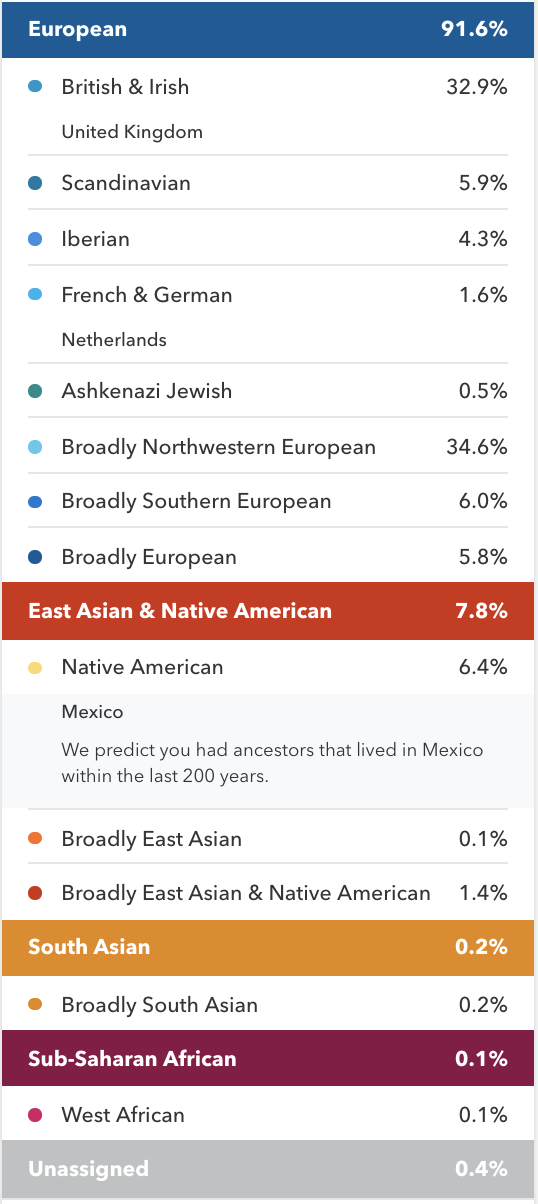
Ancestry percentage tests are also widely popular (Figure 3.5.8). Customers are genotyped and their alleles are assigned to different groups from around the world (Chapter 4 will discuss human biological variation in further detail). However, the scientific significance and potential harm of ancestry percentage tests have been called into question. For example, most alleles tested are not exclusive to one population, and populations may be defined differently depending on the testing companies. If an allele is assigned to the “Irish” population, there is a good chance that the allele may have evolved in a different cultural group or region that pre-dates the formation of the country Ireland. In other words, genetic variation often pre-dates the origins the population and geographical names of the region used by genetic testing companies. Another critique is that someone’s identity need not include biological relationships. Individuals also have the option to find and connect online with individuals with whom they share portions of their genome, which has resulted in both positive and negative outcomes. Another interesting consideration is that law enforcement is currently developing forensic techniques that involve mining DTC genomic databases for the purpose of identifying suspects linked to crimes. Regardless of these various considerations, there are now millions of individuals worldwide who have “unlocked the secrets” of their DNA, and the multi-billion dollar genomics market only continues to grow.
As you have seen in this chapter, DNA provides instructions to our cells, which results in the creation and regulation of proteins. Understanding these fundamental mechanisms is important to being able to understand how the evolutionary process works (see Chapter 4) and how humans vary from one another (see Chapters 13 and 14). It is also the advancement in genetic technologies—including ancient DNA studies, genomics, and epigenetics—that has led to new anthropological understandings about our biological relationships to other living (extant) and extinct primates. Many of these genetic discoveries will be covered in the chapters to come.
FIGURE ATTRIBUTIONS
Figure 3.5.1 Epigenetic Control (Biology 2e, Figure 16.7) by OpenStax is used under a CC BY 4.0 License.
Figure 3.5.2 “Rue” the calico cat by Hayley Mann is under a CC BY-NC 4.0 License.
Figure 3.5.3 PCR electrophoresis gel by Hayley Mann is under a CC BY-NC 4.0 License.
Figure 3.5.4 Sanger sequencing with heterozygous result by Hayley Mann is under a CC BY-NC 4.0 License.
Figure 3.5.5 DNA microarray by Guillaume Paumier (user:guillom) is used under a CC BY-SA 3.0 License.
Figure 3.5.6 Illumina Hiseq 2,000 sequencers, BGI Hong Kong sequencing room by Scotted400 is used under a CC BY 3.0 License.
Figure 3.5.7 Positive carrier result for celiac disease allele by Hayley Mann is under a CC BY-NC 4.0 License.
Figure 3.5.8 DNA ancestry percentage test results by Hayley Mann is under a CC BY-NC 4.0 License.