4.1: Nervous System Tissue
- Page ID
- 121417
This page is a draft and under active development. Please forward any questions, comments, and/or feedback to the ASCCC OERI (oeri@asccc.org).
- Differentiate the central nervous system from the peripheral nervous system
- Name the two types of cells found in nervous tissue
- Explain the basic functions of neurons and glia
- Describe the structures of a neuron
- Give an example of a type of neuron that is classified by its structure, function, or other characteristic
- Give an example of a glial cell and its function
Overview
This module presents a brief overview of the nervous system and goes into greater detail on nervous tissue cell types, including neurons (structure, types, and neurogenesis) and glia (types and functions).
As you drive into a parking lot, a skateboarder suddenly flies in front of your car across your field of vision (Figure \(\PageIndex{1}\)). You see the skateboarder in the nick of time and react immediately. You slam on the brakes and steer sharply to the right — all in the blink of an eye. You avoid a collision, but just barely. You’re shaken up but thankful that no one was hurt. How did you respond so quickly? Such rapid responses are controlled by your nervous system.
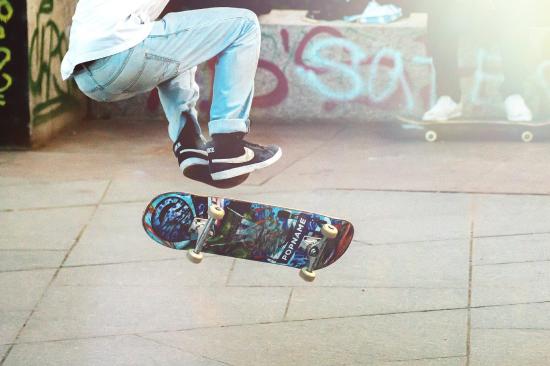
Overview of the Nervous System
The nervous system, illustrated in Figure \(\PageIndex{2}\), is the human organ system that coordinates all of the body’s voluntary and involuntary actions by transmitting electrical and chemical signals to and from different parts of the body. Specifically, the nervous system extracts information from the internal and external environments using sensory receptors. It then usually sends signals encoding this information to the brain, which processes the information to determine an appropriate response. Finally, the brain sends signals to muscles, organs, or glands to bring about the necessary response. The two main divisions of the nervous system are the central nervous system (CNS), consisting of the brain and the spinal cord, and the peripheral nervous system (PNS), which includes all other nervous tissue, such as ganglia and nerves, outside the brain and spinal cord. The CNS and PNS are covered in greater detail in separate sections. In the example above, your eyes detected the skateboarder, the information traveled to your brain, and your brain instructed your body to act to avoid a collision.
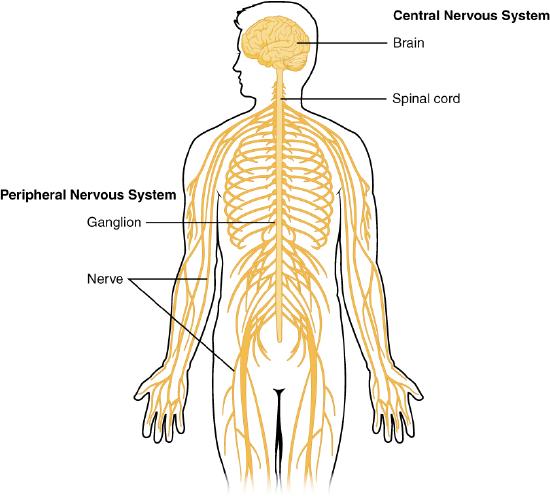
Nervous Tissue Cell Types
Nervous tissue is composed of two types of cells: neurons (also called nerve cells) and glia (also called glial cells or neuroglia), as shown in Figure \(\PageIndex{3}\). Neurons are responsible for the computation and communication that the nervous system provides. They are electrically active and release chemical signals to target cells. Glia are known to play a supporting role for nervous tissue. Ongoing research pursues an expanded role that glial cells might play in signaling, but neurons are still considered the basis of this function. Neurons are important, but without glial support they would not be able to perform their function.
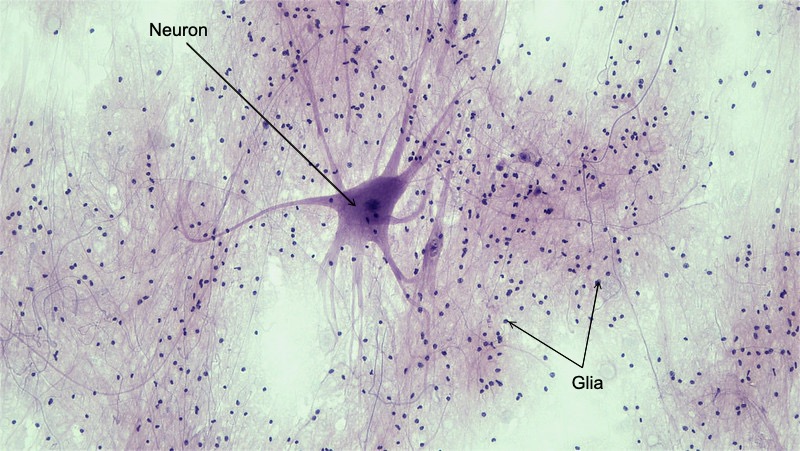
Neurons
Neurons, also called nerve cells, are electrically excitable cells that are the main functional units of the nervous system. Their function is to transmit nerve impulses. They are the only type of human cells that can carry out this function. Neurons are large cells with a high metabolic rate and they depend on a continuous and abundant supply of oxygen and glucose. Neurons are responsible for the electrical signals that communicate information about sensations, produce movements in response to those stimuli, and induce thought processes within the brain. An important part of the function of neurons is in their structure, or shape. The three-dimensional shape of these cells makes the immense numbers of connections within the nervous system possible. In the micrograph of human nervous tissue, Figure \(\PageIndex{4}\), the neon green structures in the forefront of the image are neurons.
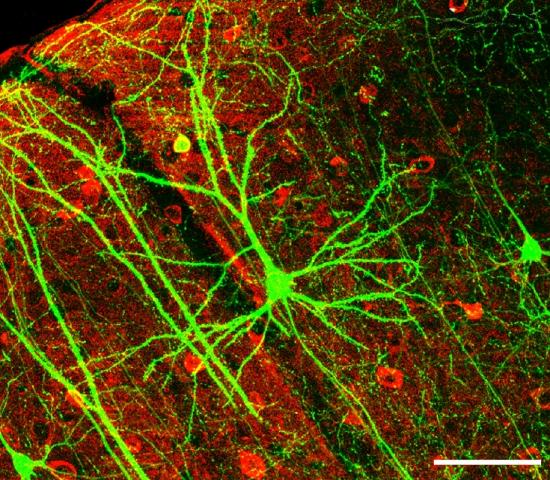
Neuron Structure
The main thing that makes neurons special and differentiates them from other cells in the body is that they have many extensions of their cell membranes, generally referred to as processes. Neurons are usually described as having one, and only one, axon—a fiber that emerges from the cell body and projects to target cells. That single axon can branch repeatedly to communicate with many target cells. It is the axon that propagates the nerve impulse (also called an action potential), which is communicated to one or more cells. The other processes of the neuron are dendrites, which receive information from other neurons across specialized areas called synapses. The dendrites are usually highly branched processes, providing locations for other neurons to communicate with the cell body. Information flows through a neuron from the dendrites, across the cell body, and down the axon.
Figure \(\PageIndex{5}\) shows the structure of a typical neuron. The main parts of a neuron are labeled in the figure and described below.
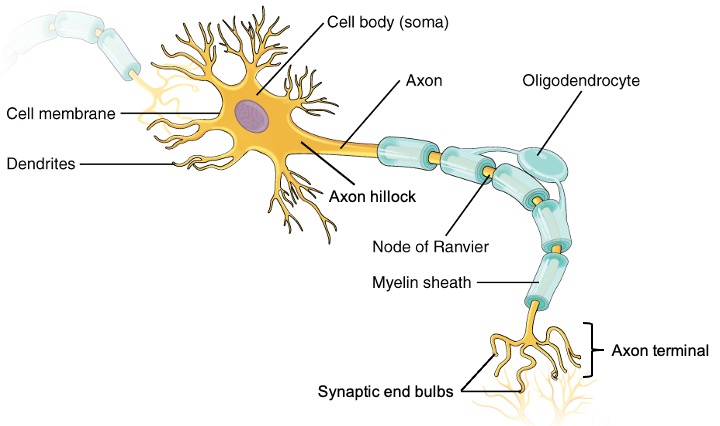
- The cell body (or soma; soma = "body") is the part of a neuron that contains the nucleus (shown as an oval structure in the center of the cell body, but not labeled) and most of the major organelles. The cell body is usually quite compact, and may not be much wider than the nucleus. The cell membrane is the structure that surrounds all the surfaces of the cell (including the dendrites and axon) and separates the inside of the cell from the outside of the cell.
- Dendrites are thin structures that are extensions of the cell body. Their function is to receive messages (excitatory and inhibitory post-synaptic potentials, EPSPs/IPSPs- see the nervous system communication chapter) from other cells and carry them to the cell body. A neuron may have many dendrites, and each dendrite may branch repeatedly to form a dendrite “tree” with more than 1,000 dendritic branches. Dendritic spines (small extensions on the surface of the dendritic branches) further increase surface area for receiving messages, allowing a given neuron to communicate with thousands of other cells.
- The axon is a long, thin extension of the cell body. It transmits nerve impulses away from the cell body and toward other cells. The axon hillock is a small bulge found at the base of motor neuron axons. The nerve impulse (or action potential) starts from the axon hillock.
- The axon branches at the end, forming the axon terminal. Branches of the axon terminal end in axon terminal buttons (also called axon endings, synaptic end bulbs, synaptic buttons/boutons, bouton terminaux, etc.) These are the points where the message is transmitted to other cells (via the release of chemicals called neurotransmitters), often to the dendrites of other neurons. A small gap called a synapse (also called a synaptic gap or synaptic cleft) is located between the end of the axon terminal and the surface of the receiving cell. An axon may branch hundreds of times, but there is never more than one axon per neuron.
- Many axons (especially the long axons of nerves in the peripheral nervous system) are covered by sections of myelin (also called the myelin sheath). The myelin sheath is composed of lipid layers that surround the axon. Myelin is a very good electrical insulator, like the plastic or rubber that encases an electrical cord. Axons that are covered by sections of myelin are called myelinated, whereas axons without myelin sheaths are called unmyelinated.
- Regularly spaced gaps between sections of myelin occur along the axon. (The gaps are actually much further apart than is shown in the figure- it is necessary to shrink the distance to fit all the structures in a diagram!) These gaps are called nodes of Ranvier, and they allow the transmission of nerve impulses along the axon. Nerve impulses jump from node to node in a process called saltatory conduction, allowing nerve impulses to travel along the axon very rapidly.
- The oligodendrocyte shown in the figure is a glial cell that produces myelin sheaths in the central nervous system (brain and spinal cord)- see the Glia section below.
Classification of Neurons
There are many neurons in the nervous system- an estimated 86 billion in the brain alone (Lent et al., 2012). And there are many different types of neurons, exhibiting a variety of structures and functions. Neurons can be classified depending on their structure, function, or other characteristics.
Structural Classification
One type of structural classification depends on the number of processes attached to the cell body: one, two or multiple (Figure \(\PageIndex{6}\)). The structures of these three different types of vertebrate neurons support their unique functions, as described below.
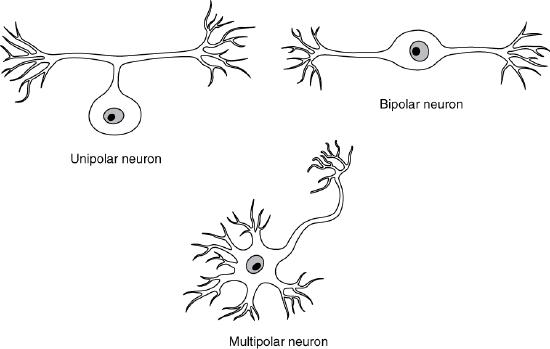
Unipolar neurons have only one process emerging from the cell. True unipolar cells are only found in invertebrate animals, so the unipolar cells in humans are more appropriately called “pseudo-unipolar” cells. (Invertebrate unipolar cells do not have dendrites.) Human unipolar cells have an axon that emerges from the cell body, but it splits so that the axon can extend along a very long distance. At one end of the axon are dendrites, and at the other end, the axon forms synaptic connections with a target. Unipolar neurons are structured in a way that is ideal for relaying information forward. Unipolar cells are exclusively sensory neurons (see functional classification below), involved in processes like transmission of physiological information from the body’s periphery (such as communicating body temperature through the spinal cord up to the brain).
Bipolar neurons have two processes which extend from each end of the cell body opposite to each other. One is the axon and the other is the dendrite. Bipolar neurons help acquire and pass sensory information to various centers in the brain, and are not very common. Bipolar cells are are involved in sensory perception such as smell (from the olfactory epithelium), and light (in the retina of the eye). The bipolar cells of the retina are an intermediate layer of neurons between the deepest layer, the visual receptors (photoreceptors, comprised of the rods and cones), and the retinal ganglion cells, the most superficial layer of retinal neurons, whose axons bundle together to form the optic nerve (see the chapter on sensory systems for more information).
Multipolar neurons are all of the neurons that are not unipolar or bipolar, and are also the most common. They have one axon (which can branch forming multiple endings) and two or more dendrites (usually many more), which allows them to communicate with many other neurons. Multipolar neurons convey sensory and motor information in the brain, spinal cord, and throughout the body. With the exception of the unipolar sensory ganglion cells, and the two specific bipolar cells mentioned above, all other neurons are multipolar. Multipolar neurons can have multiple functions and include motor neurons (see functional classification below), which carry commands from the brain and spinal cord to muscles and glands, and interneurons (see functional classification below), which constitute the majority of neurons. One of the most prominent neuron types is a pyramidal neuron, which falls under the multipolar category. As mentioned below, it gets its name from the triangular or pyramidal shape of its soma (for examples see, Furtak, Moyer, & Brown, 2007).
Functional Classification
The main functional classification depends on which function the neuron is carrying out: sensation, integration or motor. A mnemonic to remember the functional classification and which direction the nerve impulses travel (towards the CNS= afferent; away from the CNS= efferent) is "SAME" (Sensory -- Afferent and Motor -- Efferent).
Sensory (also called afferent) neurons carry nerve impulses from sensory receptors in tissues and organs to the central nervous system. They convert physical stimuli such as touch, light, and sound into nerve impulses.
Motor (also called efferent) neurons, like the one in Figure \(\PageIndex{5}\), carry nerve impulses from the central nervous system to muscles and glands. These signals then stimulate or inhibit the activity of these structures.
Interneurons carry out integrative functions (such as retrieving, processing, and storing information) and facilitate communication between sensory and motor neurons (inter = "between"). Interneurons are found in the brain and spinal cord, and their processes do not extend outside of the structure they occupy. In other words, a given interneuron will have its dendrites, cell body, and axon all contained within the brain region or spinal cord segment where it is located.
Other Classifications
Neurons can also be classified on the basis of what they look like, where they are found, who found them, what they do, or even what chemicals (neurotransmitters) they use to communicate with each other
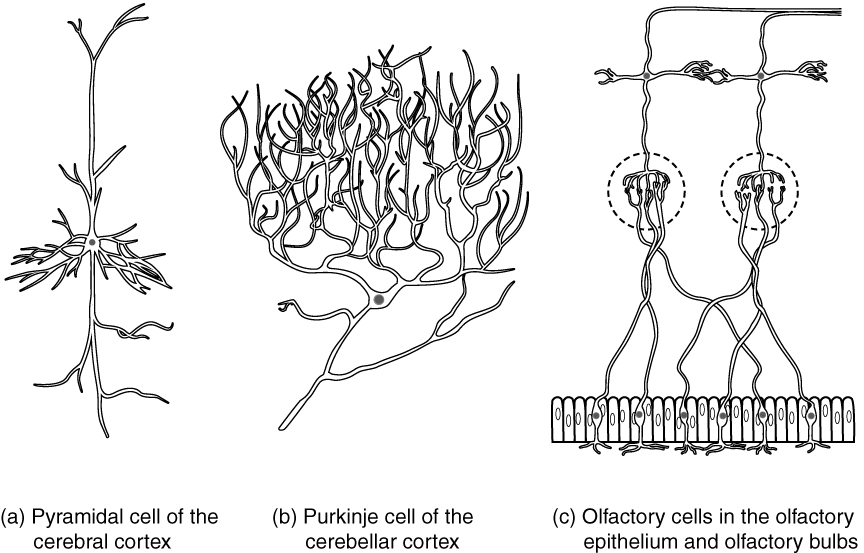
Gray Matter and White Matter
The nervous tissue in the brain and spinal cord consists of gray matter and white matter. Gray matter contains mainly the cell bodies and dendrites of neurons. It is gray only in cadavers; living gray matter is actually more pink than gray (Figure \(\PageIndex{8}\)). White matter consists mainly of myelinated axons, giving it a white color. In the brain, the gray matter is on the outside surface (the cortex) and the white matter is on the interior; in the spinal cord it is reversed- the gray matter is inside and the white matter surrounds it.
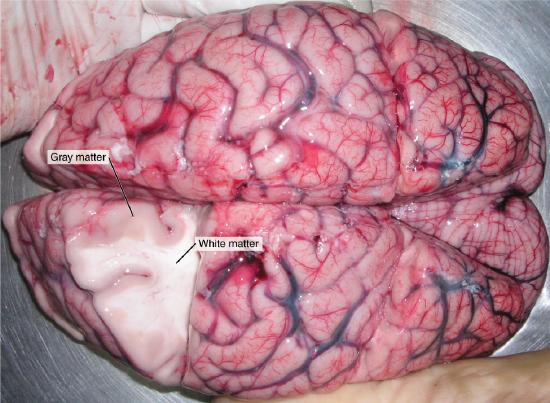
Nerves in the peripheral nervous system are also white matter. Nerves consist of long bundles of myelinated axons that extend to muscles, organs, or glands throughout the body. The axons in each nerve are bundled together like wires in a cable. Axons in nerves may be more than a meter long in an adult. The longest nerve runs from the base of the spine to the toes.
Neurogenesis
Fully differentiated neurons, with all their special structures, cannot divide and form new daughter neurons. Until recently, scientists thought that new neurons could no longer be formed after the brain developed prenatally (see the section on nervous system development). In other words, they thought that people were born with all the brain neurons they would ever have, and as neurons died, they would not be replaced. However, new evidence shows that additional neurons can form in certain areas of the brain, even in adults, from the division of undifferentiated neural stem cells that are found throughout the brain. The production of new neurons is called neurogenesis. The extent to which it can occur in adult humans is not known, but it is not likely to be very great.
Recent research indicated that new neurons formed in the amygdala of adult mice (Jhaveri at al., 2018). As the amygdala is important for emotional memory, particularly related to fearful experiences, future research may suggest new treatments for disorders such as post-traumatic stress disorder (PTSD) and depression.
If research on rats is found to apply also to humans, then sustained aerobic exercise such as running can increase neurogenesis in the adult brain, specifically in the hippocampus, a brain structure important for memory as well as learning temporally and/or spatially complex tasks. Although the research is still in the beginning stages, it suggests that exercise may actually lead to a “smarter” brain. Exercise is certainly beneficial for your body, even if it ends up not being confirmed to result in neurogenesis in humans, so it can’t hurt to get more aerobic exercise!
Glia
Glia (also called neuroglia or glial cells), are the other type of cell found in nervous tissue. They are considered to be vital supporting cells, and many functions are directed at helping neurons complete their function for communication. Although they do not participate in the communication between cells in the same fashion that neurons do, some researchers have found evidence that suggests that some glia may participate in information processing activities along with neurons (Fields & Stevens-Graham, 2002; Perea, et al., 2014). Some glial functions include digesting debris from dead neurons, carrying nutritional support from blood vessels to the neurons, and helping to regulate the ionic composition of the extracellular fluid.
The name glia comes from the Greek word that means “glue,” and was coined by the German pathologist Rudolph Virchow, who wrote in 1856: “This connective substance, which is in the brain, the spinal cord, and the special sense nerves, is a kind of glue (neuroglia) in which the nervous elements are planted.” Modern research into nervous tissue has shown that there are many deeper roles that these cells play. After his death in 1955, Albert Einstein's brain was studied by scientists worldwide—all wanting to gain insight into the anatomy of a genius. But it wasn't until the 1980s when Marian Diamond reported that Einstein had more glial cells than the average male brain (Diamond et al., 1985). Now it is clear that glia may play a more active role in brain activity, and research may reveal much more about them in the future.
One type of glial cell, radial glia, exist only during prenatal development, guiding migrating neurons to their final destinations. After this task is complete, most of these cells become neurons, but some become astrocytes or oligodendrocytes (Kalat, 2019), described below. There are six types of glial cells in the adult nervous system. Four of them are found in the CNS- astrocytes, oligodendrocytes, microglia and ependymal cells, and two are found in the PNS- satellite cells and Schwann cells. Table \(\PageIndex{1}\) outlines some common characteristics and functions of these glia.
CNS glia | PNS glia | Basic function |
---|---|---|
Astrocyte | Satellite cell | Support of the nervous tissue, form the blood-brain barrier (BBB) |
Oligodendrocyte | Schwann cell | Insulation, myelination |
Microglia | - | Immune surveillance and phagocytosis |
Ependymal cell | - | Creating cerebrospinal fluid (CSF) |
Important Functions of Some Glia
Astrocytes, named for their star-shaped appearance under the microscope (astro = “star”), have many processes extending from their main cell body (not axons or dendrites like neurons, just cell extensions). Those processes interact with neurons, blood vessels, or the connective tissue covering the CNS known as the pia mater. One important role of astrocytes is their contribution to the blood-brain barrier. The brain and spinal cord are isolated from the circulation — and most toxins or pathogens in the blood — by the blood-brain barrier, a highly selective membrane that separates the circulating blood from the extracellular fluid in the CNS. The barrier allows water, certain gases, glucose, and some other molecules needed by the brain and spinal cord to cross from the blood into the CNS while keeping out potentially harmful substances. Very little can pass through by diffusion. Most substances that cross the wall of a blood vessel into the CNS must do so through an active transport process. While this barrier protects the CNS from exposure to toxic or pathogenic substances and makes the CNS less susceptible to injury, it also keeps out the cells that could protect the brain and spinal cord from disease and damage (such as white blood cells, one of the body’s main lines of defense). Damage to the CNS is thus likely to have more serious consequences. The blood-brain barrier also causes problems with drug delivery to the CNS. Pharmaceutical companies are challenged to design drugs that can cross the blood-brain barrier as well as have an effect on the nervous system. Aside from finding efficacious substances, the means of delivery is also crucial.
Schwann cells and oligodendrocytes are the glia that produce the myelin sheath insulating axons. A single entire Schwann cell wraps around and surrounds a portion of only one axon segment in the peripheral nervous system, whereas oligodendrocytes have processes that reach out to multiple axon segments in the central nervous system. Oligodendrocyte (sometimes called just “oligo”), means “cell of a few branches” (oligo = “few”; dendro = “branches”; cyte = “cell”). Each dendritic process extends from the cell body and wraps around an axon many times to insulate it in myelin. One oligodendrocyte will provide the myelin for many axon segments- 30 to 50 (Kalat, 2019), either along the same axon or by branching out to separate axons.
The diagram (Figure \(\PageIndex{9}\)) shows several types of central nervous system cells associated with two multipolar neurons. Astrocytes are star-shaped cells with many dendrite-like projections but no axon. They are connected with the multipolar neurons and other cells in the diagram through their dendrite-like projections. Ependymal cells have a teardrop shaped cell body and a long tail that branches several times before connecting with astrocytes and the multipolar neuron. Microglial cells are small cells with rectangular bodies and many dendrite-like projections stemming from their shorter sides. The projections are so extensive that they give the microglial cell a fuzzy appearance. The oligodendrocytes have circular cell bodies with dendrite-like projections. Each projection is connected to a segment of myelin sheath on the axons of the multipolar neurons. In the diagram, the oligodendrocytes are the same color as the myelin sheath segment and are adding layers to the sheath using their projections.
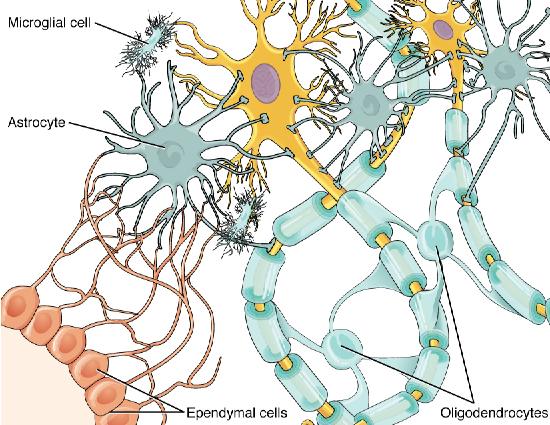
Summary
The nervous system coordinates all of the body’s voluntary and involuntary actions by transmitting electrical and chemical signals to and from different parts of the body. The two main divisions of the nervous system are the central nervous system (CNS, the brain and the spinal cord), and the peripheral nervous system (PNS, all other nervous tissue in the body).
Nervous tissue contains two major cell types, neurons and glial cells. Neurons are the cells responsible for communication through electrical signals. Glial cells are supporting cells, maintaining the environment around the neurons.
The structures that differentiate neurons from other body cells are the extensions of their cell membranes, namely one axon that projects to target cells, and one or more dendrites, which receive information from other neurons across specialized areas called synapses. The axon propagates nerve impulses (action potentials), which are communicated to one or more cells.
Neurons can be classified depending on their structure, function, or other characteristics. One structural classification is based on the number of processes the neuron has- one (unipolar), two (bipolar) or many (multipolar). One functional classification groups neurons into those that participate in sensation (sensory neurons), integration (interneurons) or motor (motor neurons) functions. Some other ways of classifying neurons include what they look like, where they are found, who found them, what they do, or what neurotransmitters they use.
The nervous tissue in the brain and spinal cord consists of gray matter and white matter. Gray matter contains the cell bodies and dendrites of neurons and white matter contains myelinated axons. Typically, neurons cannot divide to form new neurons. Recent animal research indicates that some limited neurogenesis is possible, but the extent to which this applies to adult humans is unknown.
Several types of glial cells are found in the nervous system, including astrocytes, oligodendrocytes, microglia, and ependymal cells in the CNS, and satellite cells and Schwann cells in the PNS. Astrocytes contribute to the blood-brain barrier that protects the brain. Oligodendrocytes and Schwann cells create the myelin that insulates many axons, allowing nerve impulses to travel along the axon very rapidly.
References
Note: These references are specifically those added to the content of this page by Naomi Bahm and do not include citations from the outside sources used. Refer to the text attributions to locate citations for articles from other sources.
Diamond, M.C., Scheibel, A.B., Murphy, G.M., & Harvey, T. (1985). On the brain of a scientist: Albert Einstein. Experimental Neurology, 88 (1), 198-204. https://doi.org/10.1016/0014-4886(85)90123-2. (https://www.sciencedirect.com/science/article/pii/0014488685901232)
Editors of Encyclopedia Britannica (2021). Jan Evangelista Purkinje. Encyclopedia Britannica. https://www.britannica.com/biography...lista-Purkinje. Accessed June 2, 2022.
Fields, R. D., & Stevens-Graham, B. (2002). New insights into neuron-glia communication. Science, 298 (5593), 556-562.
Jhaveri, D.J., Tedoldi, A., Hunt, S., Sullivan, R., Watts, N.R., Power, J.M., Bartlett, P.F., & Sah, P. (2018) Evidence for newly generated interneurons in the basolateral amygdala of adult mice. Molecular Psychiatry 23, 521–532. doi.org/10.1038/mp.2017.134
Lent, R., Azevedo, F.A., Andrade-Moraes, C.H., & Pinto, A.V. (2012) How many neurons do you have? Some dogmas of quantitative neuroscience under revision. European Journal of Neuroscience 35(1),1-9. doi: 10.1111/j.1460-9568.2011.07923.x. Epub 2011 Dec 13. PMID: 22151227.
Perea, G., Sur, M., & Araque, A. (2014). Neuron-glia networks: integral gear of brain function. Frontiers in cellular neuroscience, 8, 378.
Additional Resources
Interested in the effects of exercise on the brain? See Exercise and the Brain
Watch an animation of Schwann cell damage and recovery: Nerve Damage
More information on neurogenesis in the amygdala of adult mice: Emotion processing region produces new adult brain cells
Multiple sclerosis (MS) is a progressive degenerative disease that is caused by the demyelination of axons in the central nervous system. When myelin degrades, the conduction of nerve impulses along the nerve can be impaired or lost, and the nerve eventually withers. Watch this inspirational TED talk in which the speaker shares how being diagnosed with MS changed her life and led her to become an MS nurse.
Attributions
Figures:
- Skateboarder by JESHOOTS-com via Pixabay license
- Overview of nervous system by OpenStax, licensed CC BY 4.0 via Wikimedia Commons
- Labeled Nervous Tissue Smear by Chiara Mazzasette adapted from OpenStax, licensed CC BY 4.0 via Wikimedia Commons
- Interneurons of Adult Visual Cortex by Wei-Chung Allen Lee, Hayden Huang, Guoping Feng, Joshua R. Sanes, Emery N. Brown, Peter T. So, Elly Nedivi, licensed CC BY 2.5 via Wikimedia. Commons
- Neuron by Chiara Mazzasette adapted from OpenStax, licensed CC BY 4.0 via Wikimedia Commons
- Neuron Shape Classification by OpenStax is licensed under CC BY 4.0
- Other Types of Neurons by OpenStax is licensed under CC BY 4.0
- White and gray matter by OpenStax, licensed CC BY 4.0 via Wikimedia Commons
- Glial Cells of the CNS by OpenStax is licensed under CC-BY-4.0 (Human Anatomy)
Text adapted from:
- Introduction to the Nervous System by Suzanne Wakim & Mandeep Grewal, LibreTexts is licensed under CC BY-NC .
- Neurons by Suzanne Wakim & Mandeep Grewal, LibreTexts is licensed under CC BY-NC .
- Anatomy of Nervous Tissue by Whitney Menefee, Julie Jenks, Chiara Mazzasette, & Kim-Leiloni Nguyen, LibreTexts is licensed under CC BY .
- Neurons and Glial Cells by OpenStax, LibreTexts is licensed under CC BY .
- Central Nervous System by Suzanne Wakim & Mandeep Grewal, LibreTexts is licensed under CC BY-NC .
- Original material written by Kenneth A. Koenigshofer, Ph.D. is licensed under CC BY 4.0
- Neurons by Sharon Furtak, licensed CC BY-NC-SA 4.0 via Noba Project (originally curated by Kenneth Koenigshofer, as immediately above).
Changes: Text (and images) from sources 1 through 5 pieced together with some modifications, transitions and additional content added by Naomi I. Gribneau Bahm, PhD., Psychology Professor at Cosumnes River College, Sacramento, CA. Information also incorporated from content (sources 6 and 7) curated by Kenneth A. Koenigshofer, PhD., Chaffey College, specifically in the sections on Structural Classification (of Neurons) and Glia.