4.1: The Modern Synthesis and Population Genetics
- Last updated
- Save as PDF
- Page ID
- 177573
THE MODERN SYNTHESIS
It’s hard for us, with our typical human life spans of less than 100 years, to imagine all the way back, 3.8 billion years ago, to the origins of life. Scientists still study and debate how life came into being and whether it originated on Earth or in some other region of the universe (including some scientists who believe that studying evolution can reveal the complex processes that were set in motion by God or a higher power). What we do know is that a living single-celled organism was present on Earth during the early stages of our planet’s existence. This organism had the potential to reproduce by making copies of itself, just like bacteria, many amoebae, and our own living cells today. In fact, with today’s genetic and genomic technologies, we can now trace genetic lineages, or phylogenies, and determine the relationships between all of today’s living organisms—eukaryotes (animals, plants, fungi, etc.), archaea, and bacteria—on the branches of the phylogenetic tree of life (Figure 4.1.1).
Definition: origins of life
How the first living organism came into being.
Definition: phylogenies
Genetically determined family lineages.
Definition: phylogenetic tree of life
A family tree of all living organisms, based on genetic relationships.
Looking at the common sequences in modern genomes, we can even make educated guesses about what the genetic sequence of the first organism, or universal ancestor of all living things, would likely have been. Through a wondrous series of mechanisms and events, that first single-celled organism gave rise to the rich diversity of species that fill the lands, seas, and skies of our planet. This chapter explores the mechanisms by which that amazing transformation occurred and considers some of the crucial scientific experiments that shaped our current understanding of the evolutionary process.
Definition: universal ancestor
The first living organism, from which all living things are descended.
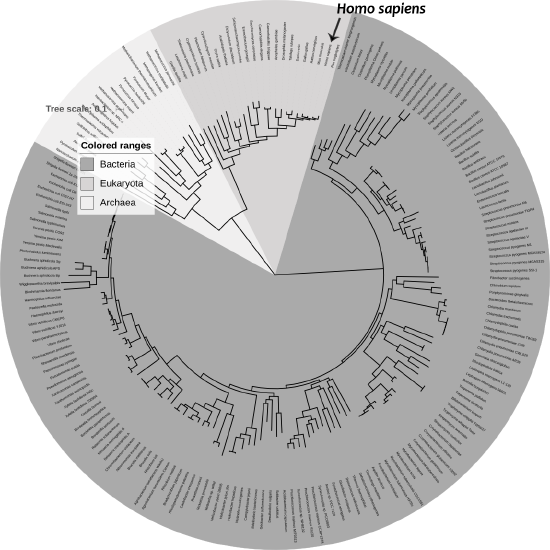
Historical Framework
When learning about biological sciences today, we always recognize the contributions of Charles Darwin and Gregor Mendel, so it may be surprising to learn that for a time, before we arrived at today’s understanding of genetics and inheritance, both Darwin’s and Mendel’s work fell out of favor. Neither Darwin’s theory of natural selection, nor Mendel’s particulate inheritance were individually sufficient to fully explain all the phenomena being observed in the natural world. It would take many decades, and many careful scientific experiments to solve the puzzle of evolution.
Rethinking Darwin
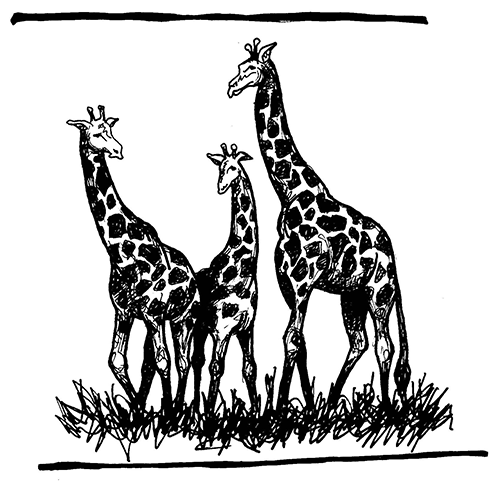
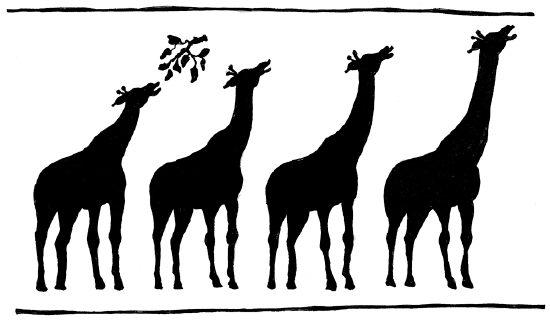
As noted in Chapter 2, Darwin’s 1859 book On the Origin of Species made a big splash; however, as other researchers began doing what scientists do—testing whether or not the concept of natural selection could consistently account for the variation seen in organisms—they began to find many exceptions. One reason for this is that, as we now know, natural selection is only one of the forces of evolution. Another challenge was a general lack of understanding about how variation is initiated and how inheritance works. Many scientists of the day subscribed to the concept known as Lamarckian inheritance, which posited that offspring would inherit characteristics that were acquired during their parents’ lifetimes (Figure 4.1.2 and 4.1.3). Darwin himself, in 1868, promoted an idea called pangenesis, which combines the Lamarckian idea of inheriting acquired characteristics with the idea that particles from different parts of the body make their way to the sex cells. Alfred Russell Wallace, evolution. Another researcher, August Weismann, also rejected the idea that acquired characteristics could be passed on. Weismann (1892) devised an experiment to directly test whether offspring inherited acquired characteristics: he cut the tails off mice, bred them, and then waited eagerly to find out if the offspring had tails. All the baby mice were born with tails intact, demonstrating Lamarckian inheritance of acquired characteristics to be incorrect (Figure 4.1.4).
Definition: Lamarckian inheritance
An early model for inheritance that predicted that offspring inherit characteristics acquired during their parents’ lifetimes. This has now been proven incorrect.
Definition: pangenesis
An early model for inheritance that combines the Lamarckian idea of inheriting acquired characteristics with the idea that particles from different parts of the body make their way to the sex cells. This has now been proven to be incorrect.
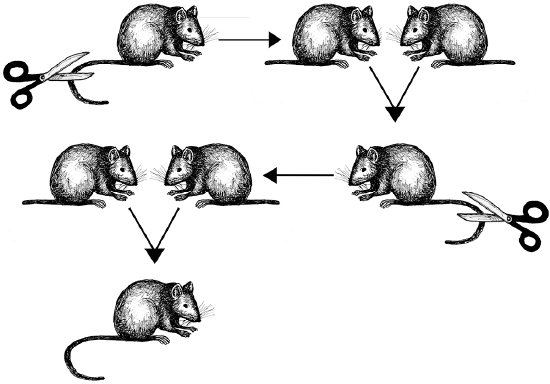
Rediscovering Mendel
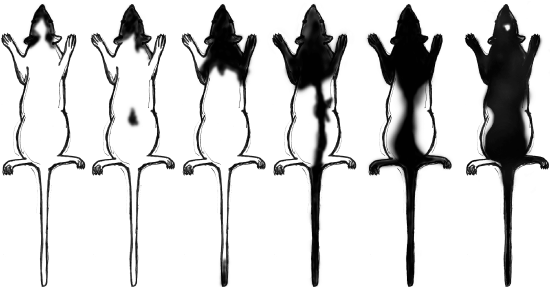
In 1900, two scientists named Hugo de Vries and Carl Correns, who were independently studying the mechanisms of inheritance, rediscovered Gregor Mendel’s work. Mendel’s pea plant experiments provided the concepts of dominant and recessive traits, which explained retention of certain characteristics in a way that Darwin’s idea, blending inheritance, didn’t. The debate that unfolded was between the Mutationists, who believed that variation was caused by mutations in distinct, inherited cells, and Biometricians, who believed that individual mutations of discrete hereditary units could never account for the continuous spectrum of variation seen in many traits. One set of experiments that helped resolve this debate was a five-year study carried out by William Castle and John Phillips on laboratory rats (Castle and Phillips 1914). The dominant coat color was the gray wild type, and the piebald or “hooded” color was recessive. He cross-bred the rats multiple ways for five generations and proved that he could achieve a continuous range of variation; in fact, he even achieved coat pattern variations that were more extreme than the original maximums of the parent groups (Figure 4.1.5).
Definition: Mutationists
A group of early biological scientists who believed that variation was caused by mutations in distinct, inherited cells.
Definition: Biometricians
A group of early biological scientists who believed that individual mutations of discrete hereditary units could never account for the continuous spectrum of variation seen in many traits.
Another scientist, Thomas Hunt Morgan, conducted studies in which he induced genetic mutations in populations of the fruit fly, Drosophila melanogaster (Figure 4.1.6). His work demonstrated that most mutations merely increased variation within populations, rather than creating new species (Morgan 1911).
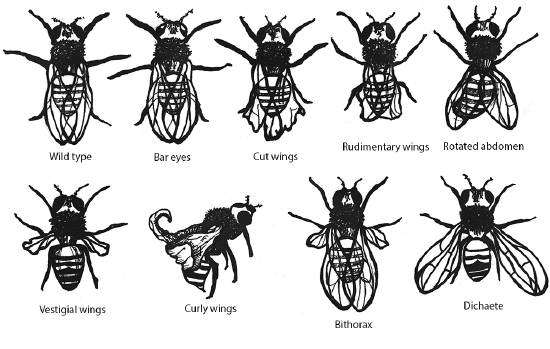
Tying It All Together
While the biggest leap forward in understanding how evolution works came with the joining (synthesis) of Darwin’s concept of natural selection with Mendel’s insights about particulate inheritance, there were some other big contributions that were crucial to making sense of the variation that was being observed. R.A. Fisher (1919) and John Burdon Sanderson Haldane (1924) developed and tested mathematical models for evolutionary change that provided the tools to study variation and became the basis for the study of population genetics. Sewall Wright (1932) and Theodosius Dobzhansky (1937) performed studies that revealed the existence of chromosomes as carriers of collections of genes. Edmund Brisco Ford (1949) conducted studies on wild butterflies that confirmed Fisher’s mathematical predictions and also led to his definition of the concept of polymorphisms to describe alternative phenotypes, or multiple forms of a trait. Ford (1942) also correctly predicted that human blood type polymorphisms were maintained in the population because they were involved in disease resistance. Julian Huxley’s 1942 book, Evolution: The Modern Synthesis, provided an easy-to-read summary of the evolutionary studies that had come before. It was with this book that the term Modern Synthesis was first used to describe the integration of Darwin’s, Mendel’s, and subsequent research into a unified theory of evolution. In appealing to the general public, Huxley’s book also found new success establishing a wide acceptance of the process of evolution.
Definition: polymorphisms
Multiple forms of a trait; alternative phenotypes within a given species.
Definition: Modern Synthesis
The integration of Darwin’s, Mendel’s, and subsequent research into a unified theory of evolution.
POPULATION GENETICS
Defining Species and Populations and the Variations Within Them
One of the major breakthroughs in understanding the mechanisms of evolutionary change came with the realization that evolution takes place at the level of populations, not within individuals. In the biological sciences, a population is defined as a group of individuals of the same species who are geographically near enough to one another that they can breed and produce new generations of individuals. Species are organisms whose individuals are capable of breeding because they are biologically and behaviorally compatible to produce viable, fertile offspring. Viable offspring are those offspring who are healthy enough to survive to adulthood. Fertile offspring can reproduce successfully to have offspring of their own. Both conditions must be met for individuals to be considered part of the same species. As you can imagine, these criteria complicate the identification of distinct species in fossilized remains of extinct populations. In those cases, we must examine how much phenotypic variation is typically found within a comparable modern-day species, and then determine whether the fossilized remains fall within the expected range of variation for a single species.
Definition: population
A group of individuals who are genetically similar enough and geographically near enough to one another that they can breed and produce new generations of individuals. A limited or restricted amount of gene flow between populations can occur due to geographical, cultural, linguistic, or environmental factors.
Definition: species
Organisms whose individuals are capable of breeding because they are biologically and behaviorally compatible to produce viable, fertile offspring.
Definition: viable offspring
Offspring that are healthy enough to survive to adulthood.
Definition: fertile offspring
Offspring that can reproduce successfully to have offspring of their own.
Some species have subpopulations that are regionally distinct. These are classified as separate subspecies because they have their own unique phenotypes and are geographically isolated from one another, but if they do happen to encounter one another, they are still capable of successful interbreeding.
Definition: subspecies
A distinct subtype of a species. Most often, this is a geographically isolated population with unique phenotypes; however, it remains biologically and behaviorally capable of interbreeding with other populations of the same species.
There are many examples of sterile hybrids that are offspring of parents from two different species. For example, horses and donkeys can breed and have offspring together. Depending on which species is the mother and which is the father, the offspring are either called mules, or hennies. Mules and hennies can live full life spans but are not able to have offspring of their own. Likewise, tigers and lions have been known to mate and have viable offspring. Again, depending on which species is the mother and which is the father, these offspring are called either ligers or tigons. Like mules and hennies, ligers and tigons are unable to reproduce. In each of these cases, the mismatched set of chromosomes that the offspring inherit still produce an adequate set of functioning genes for the hybrid offspring, but, once mixed and divided in meiosis, the gametes don’t contain the full complement of genes needed for survival in the third generation.
For the purpose of studying evolution, we recognize populations by their even smaller units: genes. Each individual, for genetic inheritance purposes, carries a collection of genes that can be passed down to future generations. For this reason, in population genetics, we think of populations as gene pools, which refers to the entire collection of genetic material in a breeding community that can be passed on from one generation to the next.
Remember, a gene is the basic unit of information that encodes the proteins needed to grow and function as a living organism. Each gene can have multiple alleles, or variants, each of which may produce a slightly different protein. For example, there are brown- or blue-pigment alleles for eye color (green is a slight variant of the brown type). The set of alleles that an individual inherits for a given gene is known as the genotype (e.g., inheriting both brown and blue eye pigments gives a genotype of Bb); while the observable traits that are produced by a genotype is known as the phenotype (e.g., a Bb individual exhibiting the dominant brown eye trait). For genes carried on our human chromosomes (our nuclear DNA), we inherit two copies of each, one from each parent. This means we may carry two of the same alleles (a homozygous genotype; eye pigment genotypes BB or bb) or two different alleles (a heterozygous genotype; eye pigment genotype Bb) for each nuclear gene. Only one of each of our alleles will get passed on to each of our children (the other will come from the child’s other parent). This means that children often inherit new genotypes and likely express unique phenotypes, compared to their parents. A common example is when two brown-eyed parents (who happen to be heterozygous for the pigment alleles) have a blue-eyed baby (genotype bb; who has inherited the recessive b alleles from both parents).
Defining Evolution
In order to understand evolution, it’s crucial to remember that evolution is always studied at the population level. Also, if a population were to stay exactly the same from one generation to the next, it would not be evolving. So evolution requires both a population of breeding individuals and some kind of a genetic change occurring within it. Thus, the simple definition of biological evolution is a change in the allele frequencies in a population over time. What do we mean by allele frequencies? Allele frequencies refer to the ratio, or percentage, of one allele (one variant of a gene) compared to the other alleles for that gene within the study population. By contrast, genotype frequencies are the ratios or percentages of the different homozygous and heterozygous genotypes in the population. Because we carry two alleles per genotype, the total count of alleles in a population will usually be exactly double the total count of genotypes in the same population (with the exception being rare cases in which an individual carries a different number of chromosomes than the typical two; e.g., Down syndrome results when a child carries three copies of Chromosome 21).
Definition: biological evolution
A change in the allele frequencies in a population over time.
Definition: allele frequencies
The ratio, or percentage, of one allele compared to the other alleles for that gene within the study population.
Definition: genotype frequencies
The ratios or percentages of the different homozygous and heterozygous genotypes in the population.
REFERENCES
Castle, W. E., and J. C. Phillips. 1914. Piebald Rats and Selection: An Experimental Test of the Effectiveness of Selection and of the Theory of Gametic Purity in Mendelian Crosses. Carnegie Institute of Washington, No. 195. Washington, DC: Carnegie Institute of Washington.
Darwin, Charles. 1859. On the Origin of Species by Means of Natural Selection, or the Preservation of Favoured Races in the Struggle for Life. London: John Murray.
Dobzhansky, Theodosius (1937). Genetics and the Origin of Species. Columbia University Biological Series. New York: Columbia University Press.
Fisher, Ronald A. (1919). “The Correlation Between Relatives on the Supposition of Mendelian Inheritance.” Transactions of the Royal Society of Edinburgh 52 (2): 399–433.
Ford, E. B. 1942. Genetics for Medical Students. London: Methuen.
Ford, E. B. 1949. Mendelism and Evolution. London: Methuen.
Haldane, J. B. S. 1924. “A Mathematical Theory of Natural and Artificial Selection (Part 1).” Transactions of the Cambridge Philosophical Society 23:19–41.
Huxley, Julian. 1942. Evolution: The Modern Synthesis. London: Allen & Unwin.
Morgan, T. H. 1911. “Random Segregation Versus Coupling in Mendelian Inheritance.” Science 34 (873): 384.
Weismann, August. 1892. Das Keimplasma: Eine Theorie der Vererbung [The Germ Plasm: A Theory of Inheritance]. Jena: Fischer.
Wright, Sewall. 1932. “The Roles of Mutation, Inbreeding, Crossbreeding, and Selection in Evolution.” Proceedings of the Sixth International Congress on Genetics 1 (6): 356–366.
FIGURE ATTRIBUTIONS
Figure 4.1.1 Tree of life SVG by Ivica Letunic: Iletunic, retraced by Mariana Ruiz Villarreal: LadyofHats, has been designated to the public domain (CC0). This item has been modified (made grayscale, rotated, labels added).
Figure 4.1.2 Lamarckian Evolution original to Explorations: An Open Invitation to Biological Anthropology by Mary Nelson is under a CC BY-NC 4.0 License.
Figure 4.1.3 Modern Synthesis original to Explorations: An Open Invitation to Biological Anthropology by Mary Nelson is under a CC BY-NC 4.0 License.
Figure 4.1.4 Weismann’s mouse-tail experiment original to Explorations: An Open Invitation to Biological Anthropology by Mary Nelson is under a CC BY-NC 4.0 License.
Figure 4.1.5 Castle’s Hooded Rat Experiment original to Explorations: An Open Invitation to Biological Anthropology by Mary Nelson is under a CC BY-NC 4.0 License.
Figure 4.1.6 Morgan’s Mutant Fruit Flies original to Explorations: An Open Invitation to Biological Anthropology by Mary Nelson is under a CC BY-NC 4.0 License.